Role and Application of Diffusion-weighted Imaging in Evaluation of Prostate Cancer
CC BY-NC-ND 4.0 · Indian J Med Paediatr Oncol 2018; 39(03): 349-354
DOI: DOI: 10.4103/ijmpo.ijmpo_41_17
Abstract
Introduction: Diffusion-weighted imaging (DWI) is an important part of magnetic resonance imaging (MRI) in the evaluation of specific organs, including the breast, kidney, liver, and prostate. Prostate cancer lesions are composed of tightly packed cells with reduced extracellular space, which can be visualized on DWIs as areas of restricted diffusion (i.e., high-signal intensity), with corresponding low-signal intensity on apparent diffusion coefficient (ADC) maps and low mean ADC value. Objective: The objective of this study is to identify the appropriate cutoff and mean ADC value to diagnose neoplastic prostatic lesions in central India. Materials and Methods: Sixty-six patients with suspected prostatic pathology were included in this study. All patients underwent MRI on a 1.5-T scanner with a phased-array body coil. MRIs were evaluated compared with the histopathological staging. Results:: The diagnostic accuracy of DWI in predicting malignancy was 39/41, i.e., 95.12%, which is the positive predicted value. The mean ADC for benign category was 1.14 with standard deviation (SD) of 0.14 while mean for prostatitis was 0.91 with SD of 0.26 and for carcinoma was 0.75 with SD of 0.19. The difference in the means was statistically highly significant. Conclusion: DWI demonstrates the restriction of diffusion and the reduction of ADC values in neoplastic tissue and improves the diagnostic accuracy in lesion characterization. This technique allows short acquisition time and provides high-contrast resolution between neoplastic and normal tissue. This technique can be a useful adjunct to the established dynamic contrast-enhanced-MRI.
Keywords
Apparent diffusion coefficient - diffusion-weighted imaging - prostate cancer - prostate pathologiesPublication History
Article published online:
17 June 2021
© 2018. Indian Society of Medical and Paediatric Oncology. This is an open access article published by Thieme under the terms of the Creative Commons Attribution-NonDerivative-NonCommercial-License, permitting copying and reproduction so long as the original work is given appropriate credit. Contents may not be used for commercial purposes, or adapted, remixed, transformed or built upon. (https://creativecommons.org/licenses/by-nc-nd/4.0/).
Thieme Medical and Scientific Publishers Pvt. Ltd.
A-12, 2nd Floor, Sector 2, Noida-201301 UP, India
Abstract
Introduction: Diffusion-weighted imaging (DWI) is an important part of magnetic resonance imaging (MRI) in the evaluation of specific organs, including the breast, kidney, liver, and prostate. Prostate cancer lesions are composed of tightly packed cells with reduced extracellular space, which can be visualized on DWIs as areas of restricted diffusion (i.e., high-signal intensity), with corresponding low-signal intensity on apparent diffusion coefficient (ADC) maps and low mean ADC value. Objective: The objective of this study is to identify the appropriate cutoff and mean ADC value to diagnose neoplastic prostatic lesions in central India. Materials and Methods: Sixty-six patients with suspected prostatic pathology were included in this study. All patients underwent MRI on a 1.5-T scanner with a phased-array body coil. MRIs were evaluated compared with the histopathological staging. Results:: The diagnostic accuracy of DWI in predicting malignancy was 39/41, i.e., 95.12%, which is the positive predicted value. The mean ADC for benign category was 1.14 with standard deviation (SD) of 0.14 while mean for prostatitis was 0.91 with SD of 0.26 and for carcinoma was 0.75 with SD of 0.19. The difference in the means was statistically highly significant. Conclusion: DWI demonstrates the restriction of diffusion and the reduction of ADC values in neoplastic tissue and improves the diagnostic accuracy in lesion characterization. This technique allows short acquisition time and provides high-contrast resolution between neoplastic and normal tissue. This technique can be a useful adjunct to the established dynamic contrast-enhanced-MRI.
Keywords
Apparent diffusion coefficient - diffusion-weighted imaging - prostate cancer - prostate pathologiesIntroduction
The application of a diffusion-weighted (DW) sequence in whole-body imaging has gained more popularity and improved the diagnostic accuracy in lesion characterization with new technical developments in magnetic resonance imaging (MRI), including echo planar imaging (EPI), and stronger gradients [1] leading to a reduction in the amount of time required for DW imaging (DWI) to <1>
Principle of diffusion-weighted imaging
DWI exploits the property of constant Brownian motion of the water molecules in tissue. This property is affected by increased cellularity, tissue organization, extracellular space, and integrity of cell membranes. DWI measures the Brownian motion of water molecules in tissues while increased cellularity restricts water diffusion. Apparent diffusion coefficient (ADC) derived from DWI demonstrates reduced ADC values and increased fractional anisotropy in prostate cancer. Free water molecules are in constant random motion, known as Brownian motion, which is related to thermal kinetic energy. In contrast, the motion of water molecules within the cellular microenvironment is impeded by their interaction with cellular compartments, including the cell wall and intracellular organelles.[2] In other words, restriction in the diffusion of water molecules is directly proportional to the degree of cellularity of the tissue. Sato et al.[3] compared the ADC values of normal and cancerous tissue in both the peripheral and transition zones at 1.5 T. In both zones, the ADC values of prostate cancer were significantly lower than those of benign tissue.[3]
This restricted diffusion is observed primarily in malignancies, [Figure 1], [Figure 2], [Figure 3], [Figure 4], [Figure 5] hypercellular metastases, and fibrosis, which contain a greater number of cells with intact cell walls than healthy tissue. Cancer tissue has higher signal intensity on high-b-value DWIs than healthy prostate tissue and will demonstrate decreased ADC values.
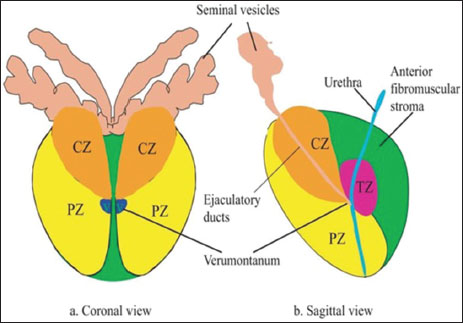
| Figure 1:Prostate-Zonal anatomy (Abbreviations: CZ – Central zone; TZ – Transition zone; PZ – Peripheral zone)
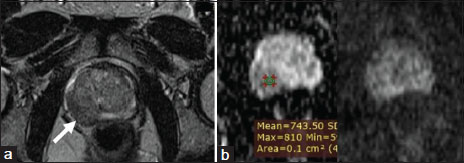
| Figure.2:Peripheral Zone Carcinoma (a) Right peripheral zone hypointensity which is equivocal (b) Focal mildly hypointense on apparent diffusion coefficient and mildly hyperintense on high b-value diffusion-weighted imaging
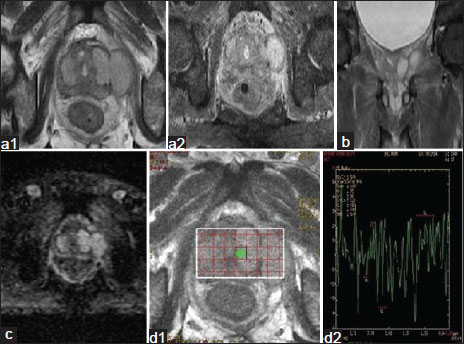
| Figure.3:Peripheral zone carcinoma. (a1 and a2) Axial image
showing iso to hypointensity involving predominantly left peripheral
and transition zone with extracapsular extension. (b) Coronal image showing extracapsular extension of the mass
lesion.
(c) Area of restricted diffusion with extracapsular extension (d1 and d2) MRS shows elevated choline and reduced
citrate
peak suggestive of prostatic carcinoma <1>
| Figure.4:MRI Urography study of Diffuse Prostatic Carcinoma showing, (a and b) sagittal T2WI images, large prostatic mass that is bulging into the urinary bladder base and pushing the large bowel loops more posteriorly with loss of fat planes between prostate and adjacent organs, (c) axial T2WI showing heterogenous isodence to hyperintense mass lesion protruding into bladder. (d) 3D MR Urography image showing hydronephrosis caused by the bladder outlet obstruction because of prostatic mass

| Figure.5:Transition zone Carcinoma. (a) T2W axial image showing ill-defined hypointensity involving predominantly left transition zone. (b) Sagittal image of the same. (c) Focal area of restricted diffusion. (d) MRS shows elevated choline and reduced citrate peak
Quantitative analysis with diffusion-weighted imaging–apparent diffusion coefficient mapping
ADC is calculated during post processing with use of at least two different b values. A more accurate ADC value can be obtained using more DWIs with different b values. The final image with different ADC values calculated for each pixel of an image is referred to as an ADC map. The regions with more restricted diffusion and therefore higher DW signal show lower ADC values.
Artifacts and pitfalls of diffusion-weighted imaging
T2 Shine-through effect
The SE sequence used in DWI is T2 weighted, and the signal intensity of the tissue depends on both the T2 signal as well as the degree of signal attenuation after applying the motion-probing gradient pulses. In tissues with very long T2 relaxation times, the strong T2signal may be mistaken for restricted diffusion. This phenomenon is known as T2 shine-through effect. V can differentiate the T2 shine through and true diffusion restriction by ADC mapping and ADC value. An area of low signal intensity on ADC (low ADC value)is seen in the true diffusion restriction and false restriction/shine through is seen as area of high signal intensity.[7]
Susceptibility artifact
Other sources of susceptibility artifact include medical devices (eg, metallic stents, surgical clips) and grafts. One way to reduce susceptibility artifact is to shorten the echo time and increase the bandwidth with image acquisition.[8]
Motion artifact
Motion artifacts due to moving organs are a source of image degradation and artifacts in DWI. Motion artifacts are more pronounced along the phase-encoding direction, where it creates “ghost” images. Increasing the speed of image acquisition, with single-shot EPI and using parallel imaging are possible ways to decrease this artifact.[9]
Materials and Methods
A diagnostic study to evaluate the role of DWI and ADC value in various prostatic pathologies, with main focus on detecting prostate cancer. Total 66 patients were informed about the nature, objective of the study, and written informed consent were taken, and Institutional Ethics Committee approval was taken beforehand. Patients with clinical suspicion of Prostate pathologies (lower urinary tract symptoms such as increased frequency of micturition, hesitancy, urgency, and hard/enlarged prostate on digital rectal examination), enlarged prostate on ultrasound abdomen and/or raised prostate-specific antigen (PSA) levels (>4 ng/ml) were included in this study. All MRI examinations were performed with a 1.5-T using the multichannel body matrix phased-array coil. The examinations were performed in T1-WI, T2-WI, DWI, and T1-weighted sequences. ADCs were calculated from transverse DWI obtained using a single-shot echo-planar imaging sequence with the following parameters: TR/TE: 3400/75 ms and b values of 0, 100, 300, 800, and 1000 s/mm.
Patient unwilling or unable (claustrophobic) to undergo MRI/magnetic resonance spectroscopy (MRS), Metallic hip implant or any other metallic implant or device that might distort local magnetic field and compromise the quality of MRI/MRS and recent biopsy were excluded from the study.
Machine used
MRI-1.5 Tesla from GE health care with body coil.
Statistical analysis
Continuous variables were summarized as mean and standard deviations (SDs) whereas nominal/categorical variables as proportions (%). A Chi-square test was used for the analysis of nominal/categorical variables. Z-test for difference between two proportions was used for comparison of nominal/categorical variables. Unpaired “t” test and ANOVA test were used for continuous variables. Diagnostic accuracy of MRI was assessed by means of sensitivity, specificity, positive predictive value, and negative predictive value. P < 0>
Results
In our study, majority of patients belonged to the age group 60–69 years constituting about 45.4%. Mean age of patients with benign pathology in our study was 60.68 ± 8.69 and with malignant pathology is 68.97 ± 7.97 years. These findings are in concordance with previous studies by Gann [10] Squillaci et al.,[17] and Costouros et al.[18]
[Table 1] gives the descriptive statistics such as mean and SD for ADC. The comparison of mean ADC was performed for each MRI-based zone across histological interpretations. In the peripheral zone (PZ), the mean ADC across interpretation groups was statistically significant with P value of 0.0211 (P < 0>
MRI based zones |
Mean±SD (n) Histopathology |
P* |
||
---|---|---|---|---|
Benign prostatic hyperplasia |
Carcinoma |
Prostatitis |
||
*Obtained using ANOVA for peripheral, t‑test for transition: S – Significant; HS – Highly significant. SD – Standard deviation; MRI – Magnetic resonance imaging |
||||
Central |
1.02±0.00 (1) |
0.74±0.17 (3) |
1.10±0.00 (1) |
- |
Peripheral |
1.07±0.23 (5) |
0.78±0.19 (21) |
0.82±0.20 (4) |
0.0211(S) |
Transition |
1.18±0.09 (13) |
0.72±0.21 (14) |
0.66±0.00 (1) |
<0> |
Peripheral and transition |
0.00 |
0.62±0.05 (3) |
0.00 |
- |
Grade |
n |
Mean±SD |
Median |
---|---|---|---|
P=0.0284 (significant) using one-way ANOVA. SD – Standard deviation |
|||
Low |
9 |
0.872±0.123 |
0.895 |
Intermediate |
14 |
0.719±0.121 |
0.718 |
High |
17 |
0.682±0.212 |
0.661 |
Histopathology |
n |
Mean±SD |
---|---|---|
P<0> |
||
BPH |
19 |
1.14±0.14 |
Prostatitis |
6 |
0.91±0.26 |
Carcinoma |
41 |
0.75±0.19 |
Histopathology |
DWI |
P* |
|
---|---|---|---|
*Obtained using Chi-square test. HS – Highly significant; DWI – Diffusion-weighted imaging |
|||
Benign |
17 |
8 <0> |
|
Malignant |
2 |
39 (HS) |
|
Sensitivity |
82.98% |
||
Specificity |
89.47% |
||
Positive predictive value |
95.12% |
||
Negative predictive value |
68.00% |
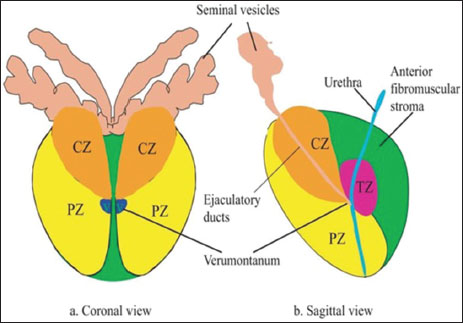
| Figure 1:Prostate-Zonal anatomy (Abbreviations: CZ – Central zone; TZ – Transition zone; PZ – Peripheral zone)
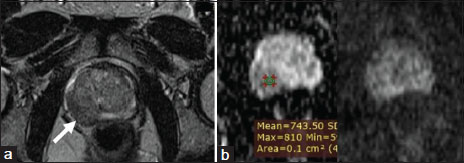
| Figure.2:Peripheral Zone Carcinoma (a) Right peripheral zone hypointensity which is equivocal (b) Focal mildly hypointense on apparent diffusion coefficient and mildly hyperintense on high b-value diffusion-weighted imaging
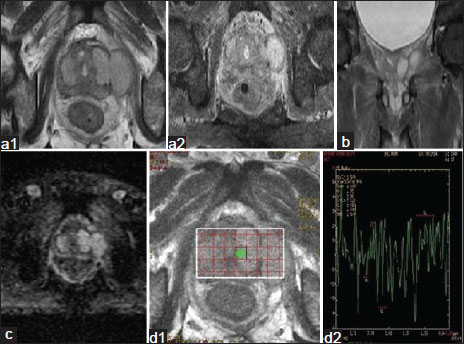
| Figure.3:Peripheral zone carcinoma. (a1 and a2) Axial image
showing iso to hypointensity involving predominantly left peripheral
and transition zone with extracapsular extension. (b) Coronal image showing extracapsular extension of the mass
lesion.
(c) Area of restricted diffusion with extracapsular extension (d1 and d2) MRS shows elevated choline and reduced
citrate
peak suggestive of prostatic carcinoma <1>
| Figure.4:MRI Urography study of Diffuse Prostatic Carcinoma showing, (a and b) sagittal T2WI images, large prostatic mass that is bulging into the urinary bladder base and pushing the large bowel loops more posteriorly with loss of fat planes between prostate and adjacent organs, (c) axial T2WI showing heterogenous isodence to hyperintense mass lesion protruding into bladder. (d) 3D MR Urography image showing hydronephrosis caused by the bladder outlet obstruction because of prostatic mass

| Figure.5:Transition zone Carcinoma. (a) T2W axial image showing ill-defined hypointensity involving predominantly left transition zone. (b) Sagittal image of the same. (c) Focal area of restricted diffusion. (d) MRS shows elevated choline and reduced citrate peak
References
- Koh DM, Collins DJ. Diffusion-weighted MRI in the body: Applications and challenges in oncology. AJR Am J Roentgenol 2007; 188: 1662-35
- Qayyum A. Diffusion-weighted imaging in the abdomen and pelvis: Concepts and applications. Radiographics 2009; 29: 1797-810
- Sato C, Naganawa S, Nakamura T, Kumada H. Miura S Differentiation of noncancerous tissue and cancer lesions by apparent diffusion coefficient values in transition and peripheral zones of the prostate. J Magn Reson Imaging 2005; 21: 258-62
- Van As N, Charles-Edwards E, Jackson A, Jhavar S, Reinsberg S, Desouza N. et al Correlation of diffusion-weighted MRI with whole mount radical prostatectomy specimens. Br J Radiol 2008; 81: 456-62
- Issa B. In vivo measurement of the apparent diffusion coefficient in normal and malignant prostatic tissues using echo-planar imaging. J Magn Reson Imaging 2002; 16: 196-200
- Shimofusa R, Fujimoto H, Akamata H, Motoori K, Yamamoto S, Ueda T. et al. Diffusion-weighted imaging of prostate cancer. J Comput Assist Tomogr 2005; 29: 149-53
- Stadnik TW, Demaerel P, Luypaert RR, Chaskis C, Van Rompaey KL, Michotte A. et al. Imaging tutorial: Differential diagnosis of bright lesions on diffusion-weighted MR images. Radiographics 2003; 23: e7
- Tartaglino LM, Flanders AE, Vinitski S, Friedman DP. Metallic artifacts on MR images of the postoperative spine: Reduction with fast spin-echo techniques. Radiology 1994; 190: 565-9
- Poustchi-Amin M, Mirowitz SA, Brown JJ, McKinstry RC, Li T. Principles and applications of echo-planar imaging: A review for the general radiologist. Radiographics 2001; 21: 767-79
- ;Gann PH. Risk factors for prostate cancer. Rev Urol 2002; 4 Suppl 5: S3-S10
- Tanimoto A, Nakashima J, Kohno H, Shinmoto H, Kuribayashi S. Prostate cancer screening: The clinical value of diffusion-weighted imaging and dynamic MR imaging in combination with T2-weighted imaging. J Magn Reson Imaging 2007; 25: 146-52
- Haider MA, van der Kwast TH, Tanguay J, Evans AJ, Hashmi AT, Lockwood G. et al. Combined T2-weighted and diffusion-weighted MRI for localization of prostate cancer. AJR Am J Roentgenol 2007; 189: 323-8
- ozlowski P, Chang SD, Goldenberg SL. et al. Diffusion-weighted MRI in prostate cancer-Comparison between single-shot fast spin echo and echo planar imaging sequences. Magn Reson Imaging 2008; 26: 72-6
- Outwater EK, Montilla-Soler JL. Imaging of prostate carcinoma. Cancer Control 2013; 20: 161-76
- Spencer JA, Chng WJ, Hudson E, Boon AP, Whelan P. Prostate specific antigen level and Gleason score in predicting the stage of newly diagnosed prostate cancer. Br J Radiol 1998; 71: 1130-5
- Singh P, Yadav MK, Singh SK, Lal A, Khandelwal N. Case series: Diffusion weighted MRI appearance in prostatic abscess. Indian J Radiol Imaging 2011; 21: 46-8
- Squillaci E, Manenti G, Mancino S, Carlani M, Di Roma M. et al. MR spectroscopy of prostate cancer Initial clinical experience. Journal of Experimental and Clinical Cancer Research: CR 2005; 24: 523-30
- Costouros NG, Coakley FV, Westphalen AC, Qayyum A, Yeh BM, Joe BN. et al. Diagnosis of prostate cancer in patients with an elevated prostate-specific antigen level: role of endorectal MRI and MR spectroscopic imaging. American Journal of Roentgenology 2007; 188: 812-6