PET/CT in paediatric malignancies - An update
CC BY-NC-ND 4.0 · Indian J Med Paediatr Oncol 2016; 37(03): 131-140
DOI: DOI: 10.4103/0971-5851.190358
Abstract
18F-fluorodeoxyglucose positron emission tomography (FDG-PET) is a well-established imaging modality in adult oncological practice. Its role in childhood malignancies needs to be discussed as paediatric malignancies differ from adults in tumor subtypes and they have different tumor biology and FDG uptake patterns. This is also compounded by smaller body mass, dosimetric restrictions, and physiological factors that can affect the FDG uptake. It calls for careful planning of the PET study, preparing the child, the parents, and expertise of nuclear physicians in reporting pediatric positron emission tomography/computed tomography (PET/CT) studies. In a broad perspective, FDG-PET/CT has been used in staging, assessment of therapy response, identifying metastases and as a follow-up tool in a wide variety of pediatric malignancies. This review outlines the role of PET/CT in childhood malignancies other than hematological malignancies such as lymphoma and leukemia.
Keywords
Central nervous system tumors - childhood malignancies - neuroblastoma - positron emission tomography/computed tomography - sarcomaPublication History
Article published online:
12 July 2021
© 2016. Indian Society of Medical and Paediatric Oncology. This is an open access article published by Thieme under the terms of the Creative Commons Attribution-NonDerivative-NonCommercial-License, permitting copying and reproduction so long as the original work is given appropriate credit. Contents may not be used for commercial purposes, or adapted, remixed, transformed or built upon. (https://creativecommons.org/licenses/by-nc-nd/4.0/.)
Thieme Medical and Scientific Publishers Pvt. Ltd.
A-12, 2nd Floor, Sector 2, Noida-201301 UP, India
Abstract
18F-fluorodeoxyglucose positron emission tomography (FDG-PET) is a well-established imaging modality in adult oncological practice. Its role in childhood malignancies needs to be discussed as paediatric malignancies differ from adults in tumor subtypes and they have different tumor biology and FDG uptake patterns. This is also compounded by smaller body mass, dosimetric restrictions, and physiological factors that can affect the FDG uptake. It calls for careful planning of the PET study, preparing the child, the parents, and expertise of nuclear physicians in reporting pediatric positron emission tomography/computed tomography (PET/CT) studies. In a broad perspective, FDG-PET/CT has been used in staging, assessment of therapy response, identifying metastases and as a follow-up tool in a wide variety of pediatric malignancies. This review outlines the role of PET/CT in childhood malignancies other than hematological malignancies such as lymphoma and leukemia.
INTRODUCTION
Cancers in children and adolescents differ from those that develop in adults. The predominant types of pediatric cancers (age 0–19 years) are leukemia (26%), brain and central nervous system (CNS) tumors (18%), and lymphoma (14%).[1,7] Some of the cancers that develop in children are rarely seen in older individuals, notably those cancers arising from embryonic cells and originating in developing tissues and organ systems. Embryonal cancers include neuroblastoma, Wilms’ tumor or nephroblastoma, medulloblastomas, rhabdomyosarcomas, and retinoblastoma. Head and neck cancers including nasopharyngeal and thyroid carcinomas are less common in children. There are not enough data to substantiate the routine use of 18F-fluorodeoxyglucose positron emission tomography (18F-FDG PET) in all childhood malignancies. Data are emerging in the use of positron emission tomography/computed tomography (PET/CT) in radiation planning for childhood head and neck cancers and in calculating gross tumor volume to accurately delineate the radiation field. Due to difficulty in performing investigations in young children and keeping in mind the extra burden of radiation, one needs to weigh the incremental benefit of PET/CT imaging in pediatric population.
Many childhood cancers are highly curable if diagnosed at an early stage. With more PET/CT centers being available, this imaging modality has been found to be useful in staging, therapy response, follow-up, and quantifying disease burden in cancer-afflicted children. Similarly, the need for further chemotherapy or external radiation can be reasonably assessed by “one stop shop” PET/CT imaging without subjecting children to unnecessary treatment-related complications. Given below is a select list of cancers where data are available for the use of PET/CT in children.
INDICATIONS FOR PET/CT IN MALIGNANCIES OTHER THAN HEMATOLOGICAL CANCERS
- Bone and soft-tissue sarcoma
- Neuroblastoma
- Brain tumors
- Other less common indications include:
- Germ cell tumors
- Hepatoblastoma
- Wilms’ tumor
- Pancreatic tumors
- Primary bone tumors.
PATIENT PREPARATION
The key to a successful PET imaging begins with appropriate patient preparation, and pediatric PET imaging is no exception to this rule. Securing a patent intravenous line, counseling the parents, and motivating children for a motionless acquisition are of paramount importance.
Oral sedation or intravenous anesthesia needs to be arranged in uncooperative children during the scan procedure. Children need to be in euglycemic status at the time of FDG injection, so 4 h fasting before FDG injection and a blood glucose level <150 mg/dL are ideal. Oral contrast is used in cooperative children. Use of intravenous contrast for CT part of PET study [1] is usually integral and may be avoided if a recent contrast study has been performed.[2] To reduce the radiation exposure, the tube current may be reasonably reduced to 25–35 mAs (pitch 1.5) in most children where the CT is used for attenuation correction and localization. Immobilization during the imaging can be achieved by wrapping sheets around the body, using sandbags or special holding devices.[3,4] Physiologic FDG uptake in thermogenic adipose tissue or brown fat is a universal problem in children and adolescents undergoing FDG-PET examination. It can introduce false-positive studies and complexity when attempting to discern physiologic activity from more ominous entities during image interpretation. This can be tackled by avoiding exposure to cold (FDG uptake can increase 12-fold during cold exposure) on the day of the study, wearing warm clothes, maintaining a warm ambient environment, and providing blankets during, immediately after FDG injection. It has been suggested that certain pharmacologic agents such as a moderate dose of oral diazepam can partly or completely block FDG uptake in brown adipose tissue. Premedication with propranolol or fentanyl sodium has also been tried. Injection fentanyl sodium is given in the dosage of 0.75–1.0 mcg/kg and it appears to be an effective alternative to moderate dose oral diazepam (0.10 mg/kg). Well-established procedural guidelines are now available for pediatric PET/CT acquisition and interpretation.[5,6,7]
DOSE RECOMMENDATION AND RADIATION DOSIMETRY
The recommended pediatric dose of 18F FDG intravenously for a PET/CT procedure through an indwelling IV cannula ranges from 0.15 to 0.30 (millicurie per kilogram) mCi/kg (5–10 MBq, megabecqueral), with a minimum dose of 1 mCi (37 MBq) and not exceeding 20 mCi (750 MBq).[8] The calculated absorbed dose is 0.24 mGy/MBq (milligray/megabecqueral) to the brain and 1.03 mGy/MBq to the urinary bladder wall. The estimated whole-body effective dose for ages of 1 year, 5 years, 10 years, and 15 years is found to be 0.095, 0.050, 0.036, and 0.025 mSv/MBq (milliSievert/megabecqueral), respectively.[9] Similarly, the radiation dose to children from CT part of PET/CT (using 80 mA and 140 kilovolts [kV]) is 3–5 mSv. The effective doses from diagnostic CT procedures are estimated to be between 1 and 10 mSv.[10]
SALIENT POINTS IN PETCT IMAGING
FDG being a simple glucose molecule provides a semiquantitative measure of glucose uptake for every lesion that is identified on a PET scan, known as standardized uptake value (SUV). This numerical value is influenced by patient's body mass (body weight and height), dose of FDG injected, and glycemic status and also may be slightly altered in the presence of oral or IV contrast inside the body close to the lesion.[11] SUV based on body surface area should be considered in pediatric patients as this SUV is a more uniform parameter than SUV based on body weight.[11]
PHYSIOLOGIC FDG DISTRIBUTION IN CHILDREN
FDG whole-body distribution in the infants differs from adults: A greater proportion of the injected activity accumulates in brain (9% vs. 7%) and less gets excreted in urine (7% vs. 20%, respectively).[8] Uptake is also variable in salivary glands, extraocular muscles, diaphragm, gastrointestinal tract, liver, skeletal muscles, myocardium, bone marrow, genitourinary tract, ovary, and testes.[12] There is preferentially more FDG uptake in the bone marrow of children than in adults due to the more extensive distribution of hematopoietic marrow. Similarly, there can be more FDG uptake in areas with lymphoid tissue (adenoids, tonsils, thymus) [Figure 1] in skeletal growth centers, particularly those of the long bone epiphyses, and thermogenic adipose tissue (also known as brown fat) [Figure 2]. This brown fat FDG uptake is often bilateral and symmetric and located in distinct locations, particularly involving the typical locations in the neck, mediastinal, para-spinal, perinephric, and para-adrenal regions. Physiologic FDG uptake in normal thymus or a chemotherapy-induced rebound thymic hyperplasia poses a diagnostic challenge in suspected lymphomas. There can be physiologic FDG excretion through renal pelvis, ureter, and urinary bladder in children,[12] which can be a hindrance in identifying pathologic uptake sites from the physiologic ones. Improper cannulation is another leading cause of false positivity showing abnormal FDG uptake in ipsilateral arm draining nodes. High bone marrow and splenic FDG uptake after the administration of hematopoietic-stimulating factors may be wrongly reported as disseminated metastasis.
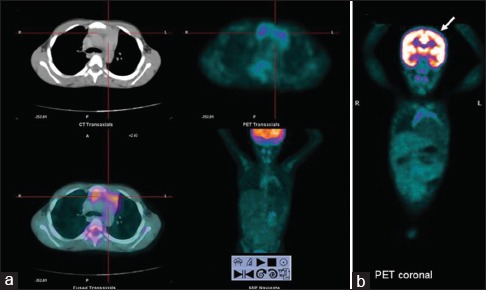
| Fig. 1 Physiologic FDG uptake in thymus (a) and brain (b)
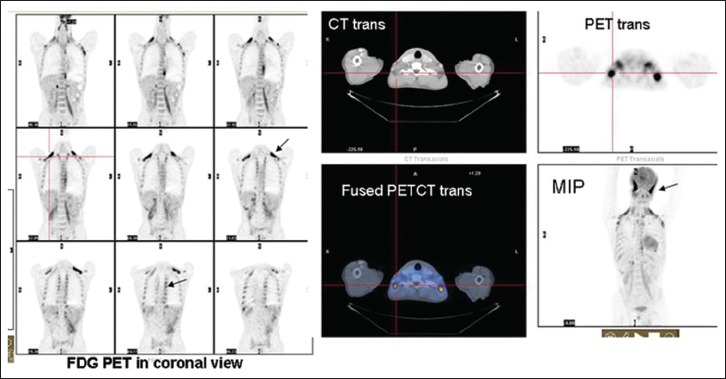
| Fig. 2 FDG PET images in coronal and transaxial and MIP images shows physiologic tracer uptake in thermogenic adipose tissue in bilateral posterior cervical, paravertebral, intercostal, and paraspinal regions. Increased fluorodeoxyglucose uptake also seen in bilateral temporalis muscles (arrow pointing to show increased FDG uptake indicating muscles overactivity)
INDICATIONS FOR PET/CT IMAGING
Bone and soft-tissue sarcoma
Sarcomas arise from mesenchymal cells and represent < 6% of all childhood cancers.[13] In children, osteosarcoma is common, followed by Ewing's sarcoma and rhabdomyosarcoma.[14] Accurate staging and identification of metastases are imperative in assessing the long-term prognosis of these patients. Bone marrow and lung metastases are common (20–30% of patients) at diagnosis. Studies have shown that FDG-PET/CT can be used in staging of bone and soft-tissue sarcomas. Charest et al. show that 93.9% of all sarcomas were identified by FDG-PET with a sensitivity of 93.7% for soft-tissue sarcomas and 94.6% for osseous sarcomas.[15] The sensitivities of the most common sarcoma with various histologies were equally good: 100% for leiomyosarcomas, 94.7% for osteosarcomas, 100% for Ewing's sarcomas, 88.9% for liposarcomas, 80.0% for synovial sarcomas, 100% for gastrointestinal stromal tumors, 87.5% for malignant peripheral nerve sheath tumors (MPNSTs), 100% for fibroblastic and myoblastic sarcomas, and 100% for malignant fibrohistiocytic tumors.[16]
Around 50% of patients with neurofibromatosis type 1 can have soft-tissue sarcoma known as MPNSTs (also called neurofibrosarcoma, neurogenic sarcoma, and malignant schwannoma).[17,18] These tumors are aggressive, likely to metastasize (40–82%) and are associated with a poor prognosis. PET imaging in a few of these sarcomas may be falsely negative [Figure 3] as FDG accumulation within a tumor is likely related to a complex interaction between the cellular energy demand and the tumoral microenvironment, apart from either fewer number of glucose transporter receptors, mitochondrial hexokinase enzyme activity, or due to small size of the lesion.[19] Correlations among tumor FDG accumulation, cell cycle, Ki-67 indices, and p53 overexpression have also been reported.[19]
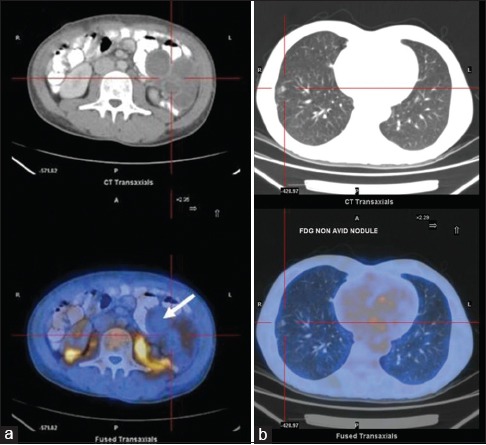
| Fig. 3 (a) Transaxial computed tomography and fused fluorodeoxyglucose positron emission tomography/computed tomography images of an adolescent patient reveal a large fluorodeoxyglucose nonavid multiseptate primary sarcomatous lesion in retromesentric plane. (b) Fluorodeoxyglucose nonavid metastatic lung nodules in the same patient
PET also serves as a robust marker for therapy response evaluation in children. Initially, this was more subjective as it was based on anatomical imaging modalities by measuring the decrease in size of lesions posttherapy called Response Evaluation Criteria in Solid Tumors (RECIST criteria).[19] Nowadays, functional imaging (PET) based response evaluation is in vogue called PET-based Response Evaluation Criteria in Solid Tumors criteria,[19,20] which is more dependable and robust.
Neuroblastoma
Neuroblastoma is the most common extracranial solid malignancy, affecting children between 20 and 30 months of age.[21] Adrenal glands, paravertebral and presacral sympathetic chain, organ of Zuckerkandl, posterior mediastinal sympathetic ganglia, and cervical sympathetic plexuses are sites that may be involved with this cancer.[21] Neuroblastoma can present with symptoms, which may be in the abdominal swelling, or due to its related mass effects such as lower limb weakness from spinal cord compression or difficulty in breathing due to an enlarged liver. Symptoms can also be caused by metastatic disease, for example, skeletal metastases leading to bony pain, orbital wall metastases presenting as Panda sign or Raccoon eyes (due to orbital ecchymoses causing darkening of periorbital tissues). Less than 2% of the patients present with paraneoplastic syndromes such as profuse diarrhea (due to secretion of vasoactive intestinal peptide) or opsoclonus-myoclonus-ataxia. Usually, anatomical localization of primary neuroblastoma is achieved with magnetic resonance imaging (MRI) or computed tomography (CT).
Functional information is best achieved using SPECT (Single photon emission computed tomography) or PET tracers. SPECT tracers include 131I-labeled metaiodobenzylguanidine (MIBG), indium (111In)-labeled pentetreotide scintigraphy while PET tracers comprise 18F FDG or 68Ga-labeled DOTA peptide SSTR somatostatin receptor) agent.[22] The treatment and outcome of neuroblastoma depend on risk assessment and stage of the disease. Thus, staging and risk stratification are important. Histopathological confirmation is mandatory in a case of suspected neuroblastoma and can be obtained from a biopsy of the primary tumor. A biopsy can, however, be avoided if bone marrow aspiration shows tumor cells in a patient with elevated urine or serum catecholamine levels. Bone is the most common site of metastasis in neuroblastoma, producing marrow or cortical lesions. Although CT scan and MRI can detect skeletal metastases, imaging investigation of choice is an MIBG scan followed by FDG PET/CT and Gallium DOTA peptide SSTR PETCT scans. The other common sites of metastases are liver and skin, especially in infants. Liver involvement in neuroblastoma can be in the form of focal lesions or diffuse infiltration causing hepatomegaly and respiratory distress. Lung and CNS metastases are extremely rare and show nonspecific and varied appearances.
Studies have compared the uptake of MIBG, FDG, and (99mTc MDP) bone scan in children with metastatic neuroblastoma, and it has been found that lesions equally concentrate one or more of these tracers pre- and post-therapy,[22] hence useful to monitor therapy response [Figure 4]. MIBG imaging was overall considered more specific and superior to FDG-PET, particularly in the delineation of residual disease. However, FDG-PET imaging can be completed early whereas MIBG imaging is performed 2–3 days later. High-resolution images can be acquired with FDG-PET/CT with excellent anatomical delineation. No thyroid blockade or withholding of drugs is advised. Two of the limitations of FDG-PET are its low sensitivity to identify bone marrow involvement [22] and not somatostatin receptor (SSTR) dependent. Therefore, selection of patients for lutetium DOTATATE therapy is not possible unless 68Ga DOTA peptide imaging is performed.
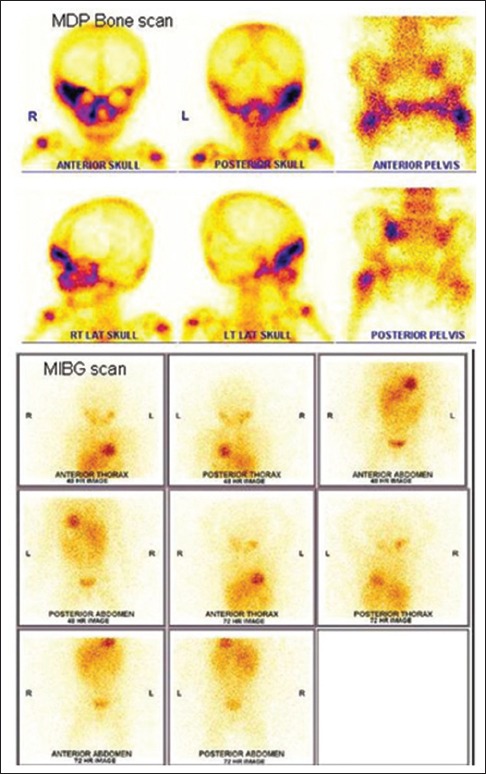
| Fig. 4 99mTechnetium methylene diphosphonate bone and 131I MIBG scan in a 16-month-old child with Stage IV neuroblastoma. Bone scan reveals multiple sites of bone metastases (right supra orbital and zygomatic bones, right maxilla, left sacro iliac joint, and left femoral trochanter) while metaiodobenzyl guanidine scan is negative
SSTR imaging using 68Gallium shows high efficacy for whole-body imaging, but there are some limitations in organs with higher physiological uptake (e.g. liver) and in terms of detection of small lesions due to the suboptimal physical resolution of the isotopes used. More recently, the development of SST-analogs radiolabeled with 68Ga for PET imaging such as [68Ga-DOTA 0-Tyr 3] octreotide (68Ga-DOTATOC, 68Ga-edotreotide), [68Ga-DOTA 0-1NaI 3] octreotide (68Ga-DOTANOC), and [68Ga-DOTA 0-Tyr 3] octreotate (68Ga-DOTATATE) has brought clear advantages as compared to radiolabeled SST-analogs scintigraphy offering a higher spatial resolution and improving pharmacokinetics. Although 68Ga-DOTATOC, 68Ga-DOTANOC, and 68Ga-DOTATATE can all bind to SSTR subtype 2, they have different affinity profiles for the other SSTR subtypes. In particular, 68Ga-DOTANOC also shows a good affinity for SSTR subtypes 3 and 5; 68Ga-DOTATOC also binds to SSTR5 (although with lower affinity than DOTANOC) while 68Ga-DOTATATE has a predominant affinity for SSTR2. Studies more recently have found that 68Ga labeled DOTA Lanreotide is useful for a broader range of SSTR subtypes. The dosimetric data measured for the whole body and specific organs using 68Ga-DOTATATE have been published recently. Although the organ doses and effective doses for 68Ga-DOTATATE and 68Ga-DOTATOC are similar (though 68Ga-DOTATOC is slightly lower), the reported dosimetry of 68Ga-DOTANOC is the lowest. Importantly, the effective dose per megabecquerel for 68Ga-labeled SST-analogs is approximately 3–5 times lower than for 111In-DTPA-octreotide, resulting in an additional advantage of PET tracers compared to radiolabeled SST-analogs scintigraphy. Date using 68Ga-DOTATATE demonstrates that this radiopharmaceutical is safe and both organ-specific and effective dose exposures are acceptable.
The primary role of FDG-PET lies in identifying those neuroblastomas and non-SSTR tumors that do not demonstrate MIBG uptake [Figure 5]. Newer agents such as 11Carbon labeled hydroxyephedrine (11C-HED) and 11C epinephrine PET have been tried. A recent study reported a greater sensitivity for 11C-HED PET than that for 123I MIBG (99% versus 93%).[23] However, cost and availability of such short-lived isotopes are a deterrent. Compounds labeled with 18F, such as fluoro norepinephrine, fluoro metaraminol, and fluoro dopamine, have an advantage over 11C labeled tracers due to their longer half-life (110 min). MIBG labeled with fluorine and 124I are also described.[24]
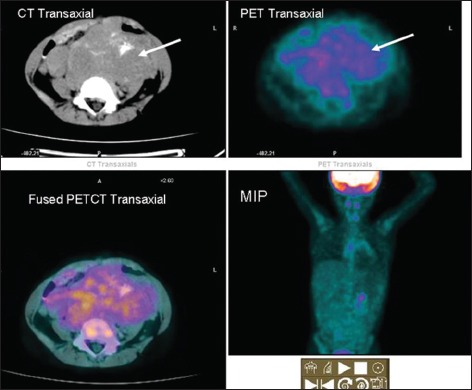
| Fig. 5 Heterogenous mildly increased fluorodeoxyglucose noted in a large primary neuroblastoma with specks of intratumoral calcification
Brain tumors
Brain tumor accounts for 21.2% of all childhood cancers.[25] Astrocytoma, the most common type of brain tumor, accounts for 35% of CNS tumors in children.[25] Childhood brain tumors differ from adult tumors in epidemiology, histologic features, and response to treatment.[25] PET has clearly defined roles in primary brain tumor imaging. High-grade gliomas have been shown to have more FDG uptake compared with low-grade or well-differentiated neoplasms, and FDG-PET can be useful in making a distinction between low- and high-grade gliomas. Using the same principle, FDG-PET is also used for prognostication of brain tumors. It is also used to identify the exact site for biopsy in a heterogeneous tumor or tumor with surrounding edema [Figure 6]. The other indication of identifying radiation necrosis versus recurrent gliomas is slightly complicated. During the early postradiation period (up to 6 months), high concentration of inflammatory cells at the site of radiation can produce high uptake of FDG mimicking recurrence. Although the sensitivity of FDG-PET to detect recurrence from radiation-induced necrosis is very high (86%), the specificity is around 40% only, making the distinction often difficult. To overcome this issue, coregistration of PET with MRI or using dual time point imaging (delayed imaging after 2 h) may be useful.

| Fig. 6 FDG PET/CT images in a 15-year-old girl suspected to have recurrent glioma. Fused FDG PET/CT transaxial images clearly demarcate the tumor site from surrounding edema
It is often difficult from MRI alone to delineate the exact extent of tumor from surrounding edema due to radiation-induced changes, even with contrast enhancement.[26] A few limitations of FDG-PET as a cerebral imaging agent are that normal brain tissue has high physiologic glucose metabolic rate producing a high FDG uptake which may mask smaller lesions. Another issue is in the detection of tumors with only modest increases in glucose metabolism, such as low-grade tumors which may be difficult to interpret.[27,28] Many of the brain tumors studied using FDG have been performed in adults.[29] There are no enough data on children.
The other important indication of PET in brain tumor is for response evaluation. Historically, tumor response had been measured using the MacDonald criteria, originally published in 1990 which is based on MRI. It provides an objective assessment of tumor response depending on the size of the enhancing tumor area, clinical status, and use of corticosteroids. The Response Assessment in Neuro-oncology working group has recently outlined a proposal for updating the Response Assessment Criteria for High-grade Gliomas which is again based on MRI. It has been emphasized to be careful in diagnosing recurrence in the first 3 months after radiation for high-grade glioma as pseudoprogression may present after 3 months following completion of radiation, showing larger areas of enhancement on pre- and post-therapy MRI. Several other agents for PET imaging are being developed, which can overcome certain limitations of FDG, and provide new information on the biologic, metabolic and molecular characteristics of malignant tumors, and aid in the diagnosis, optimal therapeutic approach, prognostic assessment and therapy monitoring in cancer patients.[30,31,32] Studies have compared uptakes of 18F fluoroethyl tyrosine (FET), FDG, and 18F-choline (FCH) in acute cerebral-radiation injury lesions and acute cryolesions (disruption of blood–brain barrier) in rat.[33] Both FDG and FCH accumulated in macrophages, a common inflammatory mediator in radiation necrosis. FET uptake was absent in macrophages. Moreover, FET uptake ratio in radiation necrosis versus normal cortex was much lower than that of FDG and FCH, suggesting FET as promising radiotracer for differentiating radiation necrosis from tumor recurrence.[32] Another group of promising tracers includes amino acid radiotracers, such as 11C-methionine, 18F-fluoro-L-phenylalanince (18F-FDOPA), and 18F-fluoroethyl-L-thyrosin (18F-FET). These molecules actively get transported into the cells regardless of disruption of the blood–brain barrier; therefore, they are able to show uptake within both low- and high-grade gliomas. FET is unique in the degree to which there is uptake in the setting of a disrupted blood–brain barrier, which makes it very useful in determining the grade of brain tumors since higher-grade cancers are associated with more disruption of the blood–brain barrier. Similar results are seen with 18F DOPA PET.[34] Amino acid radiotracers generally have a higher specificity compared with other radiotracers for distinguishing radionecrosis from tumor recurrence with reported specificity of 100% for 11C-methionine, 93.5% for 18F-FET, and 86% for 18F-FDOPA.
Methionine PET is generally false negative in cases of intermediate oligodendroglioma, metastatic tumor, chordoma, and cystic ganglion. Combined FDG with amino acid PET can serve as a guide to biopsy in brain tumor patients.[32]
FDG-PET imaging also has prognostic significance; high-FDG uptake in a previously known low-grade CNS tumor establishes the diagnosis of anaplastic transformation.[35] Coregistration of FDG-PET images with MRI greatly improves the performance of FDG-PET.[36] FLT has found to be a good prognostic marker to monitor treatment response in adults. Its uptake correlates with thymidine kinase-1 activity and therefore has a relation to proliferation index Ki-67.[37,38]
Positron emission tomography in germ cell tumor
Testicular cancer is now the most frequent malignancy among men between 20 and 40 years. Germ cell tumors are categorized into pure seminomas (40%) and into the heterogeneous group of nonseminomatous tumors comprising teratoma, chorionic carcinoma, embryonal and mixed or combination tumors. Metastatic spread is found in 70% of nonseminomatous tumors and 30% of seminomas at the time of diagnosis. They occur as painless testicular/ovarian swelling in young adults, and assessment of disease extent must be performed before initiating therapy. Diagnosis of metastatic spread is usually made by CT of the abdomen and chest and/or elevated tumor markers (human chorionic gonadotropin, alpha fetoprotein, and lactate dehydrogenase). CT-based clinical staging has been shown to have a false-negative rate. The European Germ Cell Cancer Consensus Group recommends that tumor node metastasis staging is used [39,40] and that patients are categorized using the International Germ Cell Cancer Collaborative Group classification which stratifies patients into good, intermediate, and poor prognostic groups. This latter classification is based on histology, location of primary tumor and metastases, and levels of serum markers. Due to excellent cure rates in early testicular cancer, therapy-induced morbidity (disturbances of ejaculation, infertility, surgical complications, and induction of secondary tumors) has become an important matter. The development of better noninvasive imaging techniques would be a key to a more individualized therapy. PET/CT has been found to be useful in staging the disease and also to assess response to therapy. Another problematic issue is the occurrence of indeterminate residual masses in CT after completion of chemotherapy in 15–75% of patients. Decision on the need for surgical resection of the mass or further chemoradiation is essential. There is histological proof showing necrosis/fibrosis in 40–50% of the cases, followed by differentiated teratoma (12–40%) and persistent viable malignancy in 20–40%.[40] Although teratomas should be resected because tumor progression may occur, approximately 40% of patients with residual masses after chemotherapy would not need laparotomy if viable residual tumors could be excluded noninvasively.[40] Hence, FDG-PET/CT has been used successfully for staging and assessing therapy response in various malignancies including germ cell tumors.
Positron emission tomography in hepatoblastoma and liver secondaries
Malignant liver tumors account for approximately 1% of all pediatric malignancies.[41] Hepatoblastoma, an embryonal tumor, accounts for two-thirds of malignant liver tumors in children. Ninety percent of children with hepatoblastoma have elevated alpha fetoprotein level and the remaining 10% of cases tend to be more aggressive.[42] Hepatoblastoma usually presents as large multinodular liver masses with a propensity to metastasize to lungs and contiguous tissues [43] [Figure 7]. Curative treatment is complete gross resection of the primary tumor. Complete resection of this tumor is possible in 30–40% of cases. The prognosis is poor; only 30% of children are long-term survivors.[44] Alternatively, neoadjuvant chemotherapy is advised to render tumors smaller, better demarcated, and more likely to be completely resected. Furthermore, liver transplantation has shown to be a good option in children with unresectable hepatoblastoma and without demonstrable metastatic disease after neoadjuvant chemotherapy.[45] 11Choline and 18FDG-PET have been used in these patients. Hepatoblastoma, being aggressive tumors, tends to exhibit increased glycolytic activity; however, well-differentiated cancers may be FDG less or nonavid. PET/CT is used in the follow-up period in patients showing a rising level of serum alpha fetoprotein – An indicator of tumor recurrence. Sironi et al.[46] studied nine treated cases of hepatoblastoma with suspected recurrence. FDG-PET/CT was performed in all the children. Biopsy was performed in 8/9 cases. Confidence interval (CI) and PET/CT resulted to be concordant in 5/9 patients (CI identified recurrence of disease, but 18F-FDG PET/CT provided a better definition of disease extent); in 4/9 cases, CI diagnostic information resulted in negative findings, whereas PET/CT correctly detected recurrence of disease. 18F-FDG-PET/CT showed an agreement of 100% (8/8), with fine-needle aspiration biopsy results. They concluded that FDG-PET/CT could provide an additional means of evaluating hepatoblastoma patients after surgical approach (liver transplantation or hepatectomy) in the detecting sites of recurrence. Most of the data have focused on the usefulness of PET in the detection of recurrence of hepatoblastoma, even in a transplanted liver.
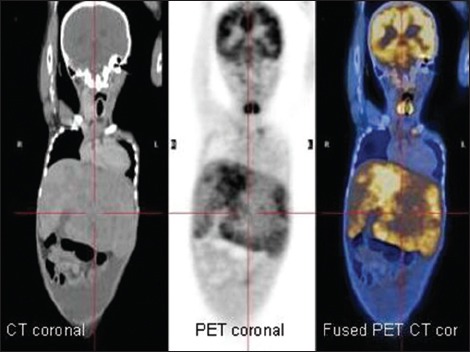
| Fig. 7 Fluorodeoxyglucose positron emission tomography/computed tomography images of a 5-year-old child with histologically proven hepatoblastoma showing variable fluorodeoxyglucose uptake (coronal sections)
There are potential limitations of 18F-FDG PET/CT in liver cancers which include poor spatial resolution of lesions (PET cannot resolve lesion < 4 mm in size), and physiologic FDG uptake in hepatocytes can mask smaller lesions.[47] The minimal mitotic activity of some of the histological subtypes such as the pure fetal subtype may demonstrate lower FDG avidity than other more unfavorable hepatoblastoma histotypes, leading to false-negative PET studies.[48]
The other liver metastatic deposits that can be encountered in children, although rare, may occur from neuroblastoma, Wilms’ tumor or nephroblastoma,[48] osteogenic sarcoma, malignant gastric epithelial tumor, and desmoplastic small round cell tumor; FDG-PET had high sensitivity and specificity for metastatic liver lesions in pediatric patients as had been demonstrated in adults.[49]
Positron emission tomography in Wilms’ tumor
Wilms’ tumor is the most common renal malignancy of childhood and is predominantly found in younger children. It is rarely encountered after the age of 5 years. Bilateral renal involvement occurs in approximately 5% of all cases. Extrarenal sites of Wilms’ tumor may include the retroperitoneum, inguinal canal, endocervix, uterus, epididymis, and sigmoid mesocolon.[50] An asymptomatic abdominal mass is the typical mode of presentation. Nephrectomy with adjuvant chemotherapy is the treatment of choice. Radiation therapy is used in selected cases when resection is incomplete. Radiography, sonography, CT, and MRI are commonly used for anatomical staging and detection of metastases. Studies have described the functional imaging of Wilms’ tumor with FDG, but a definite role for FDG-PET in Wilms’ tumor has not been established. Normal excretion of FDG through the kidney is also a limiting factor. However, careful correlation with anatomical cross-sectional imaging usually allows distinction of tumor uptake from normal renal FDG excretion. FDG-PET is found to be most useful in identifying active tumor in residual masses that persist after radiation, chemotherapy, or both and for evaluating the effects of treatment on metastatic disease. Wilms’ tumors appear to concentrate FDG, a feature that might prove clinically useful. Shulkin et al.[50] examined three patients with known or suspected Wilms’ tumor who underwent imaging with FDG-PET, and in all three patients, the results of the PET scans influenced the therapeutic decisions. The investigators concluded that FDG-PET scanning might be useful for managing Wilms’ tumors in selected patients. Larger series need to be performed to clearly define the role of FDG-PET/CT in such tumors.
Positron emission tomography in pancreatic tumors
Pancreatic tumors are of endocrine (insulinomas, gastrinomas)[51] or nonendocrine origin (pancreatoblastomas, pancreatic adenocarcinomas, cystadenomas, and rhabdomyosarcomas). Insulinomas lead to congenital hyperinsulinism, which is the most common cause of persistent hypoglycemia in infancy.[52] Hypoglycemia accompanied by high insulin level or gastric ulcers (Zollinger–Ellison syndrome) indicates the possibility of a pancreatic tumor.[53] Pancreatic islets have been shown to take up L-dopa and convert it to dopamine.[54] Thus, 18F DOPA-PET has been proved to be effective in distinguishing focal and diffuse disease with a sensitivity, specificity, and accuracy of 94%, 100%, and 75%, respectively.[55] Studies proved that the results of 18F DOPA-PET were better with hepatic vein insulin sampling and transhepatic portal venous insulin sampling, in differentiating focal from diffuse disease of the pancreas and identifying the location of the focal lesion as compared to arterial calcium stimulation.[55] Drug can interfere with F-DOPA metabolism and needs to be withdrawn 24 h before the scan. Some centers premedicate patients with carbidopa (100–200 mg 1 h before the injection). Special preparation has been described for children suspected to have congenital hyperinsulinism. Diazoxide, octreotide, and glucagon have to be stopped for 2 days, fasting for 6 h, and a glucose infusion is given to maintain euglycemia. In this case, the use of carbidopa is not recommended.[52]
Positron emission tomography in bone tumors
Osteosarcoma and Ewing's sarcoma are the most common primary bone malignancies in children. MRI identifies the extent of primary lesion in osteosarcoma and Ewing's sarcoma in bone and soft tissue. However, signal abnormalities caused by peritumoral edema can result in an overestimation of tumor extension.[56] Bone scintigraphy has been used primarily to detect osseous metastases of these tumors at diagnosis and during follow-up. Studies have shown that FDG-PET may play an important role in assessing the extent of disease, monitoring the response to therapy, and predicting the long-term outcome after therapy.[57] FDG-PET may be superior for detecting osseous metastases from Ewing's sarcoma but may be less sensitive for those from osteosarcoma.[57] Other potential role is in assessing patients with suspected or known pulmonary metastasis, which is particularly common with osteosarcoma. A recent retrospective study analyzed usefulness of PET in patients who had osteosarcoma. They found out that PET not only detected metastases in 22% of patients with 67% of those harboring disease outside the lung but also upstaged 7% of patients to Stage IV.[57]
PET/CT is recommended for the following childhood malignancies (other than hematological malignancies):[58]
- To identify site of unknown primary malignancy
- Staging of sarcoma
- Germ cell tumors (especially when tumor marker is negative, and there is retroperitoneal lymphadenopathy or mediastinal primary)
- Patients with hepatoblastoma requiring liver transplant
- Nephron-sparing surgery in children with synchronous Wilms’ tumor planned for bilateral renal surgery
- Stage III neuroblastoma after initial chemotherapy (if further chemotherapy is being considered to further reduce tumor burden)
- Mutilating surgery in children with sarcoma
- MIBG negative or residual neuroblastoma
- Soft-tissue sarcoma
- In children where accurate biopsy is essential (e.g. heterogeneous tumors where treatment may be determined by highest grade of tumor as in ganglioneuroma vs. ganglioblastoma, necrotic Wilms’ tumor)
- Children scheduled for radiation therapy planning (gross tumor volume calculation) in head and neck including nasopharyngeal carcinomas.
CONCLUSION
PET/CT is becoming increasingly important imaging tool in the noninvasive evaluation and monitoring of children with known or suspected malignant diseases. In children, FDG uptake patterns vary when compared with adults and identifying physiologic pattern from a pathologic one need experience and better image coregistration with CT. PET/CT is a noninvasive imaging tool which is a one stop shop for malignancy evaluation in adult and pediatric population. With the availability of higher end PET/CT scanners, faster acquisition and lesser radiation exposure have made this investigation more patient friendly and productive. The overexpression of SSTR is easily diagnosed using 68Ga DOTA PET and its overexpression has been reported that in most high-grade gliomas and neuroblastoma, it may be an interesting target for peptide receptor therapy.
Financial support and sponsorship
Nil.
Conflicts of interest
There are no conflicts of interest.
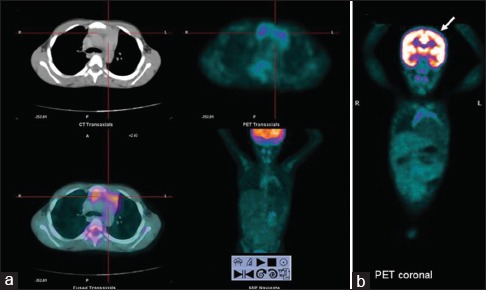
| Fig. 1 Physiologic FDG uptake in thymus (a) and brain (b)
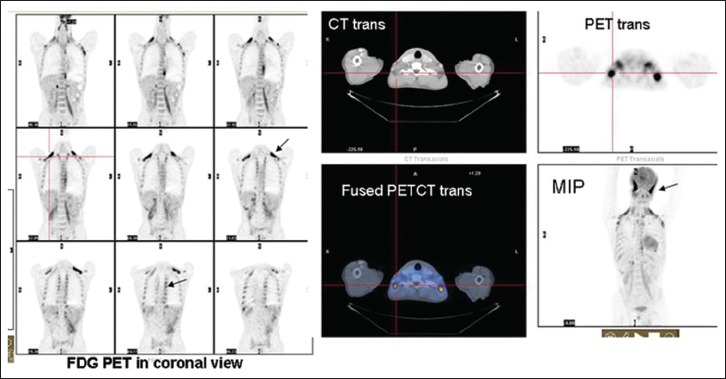
| Fig. 2 FDG PET images in coronal and transaxial and MIP images shows physiologic tracer uptake in thermogenic adipose tissue in bilateral posterior cervical, paravertebral, intercostal, and paraspinal regions. Increased fluorodeoxyglucose uptake also seen in bilateral temporalis muscles (arrow pointing to show increased FDG uptake indicating muscles overactivity)
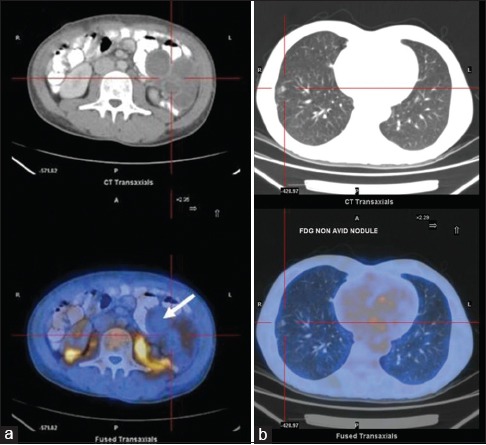
| Fig. 3 (a) Transaxial computed tomography and fused fluorodeoxyglucose positron emission tomography/computed tomography images of an adolescent patient reveal a large fluorodeoxyglucose nonavid multiseptate primary sarcomatous lesion in retromesentric plane. (b) Fluorodeoxyglucose nonavid metastatic lung nodules in the same patient
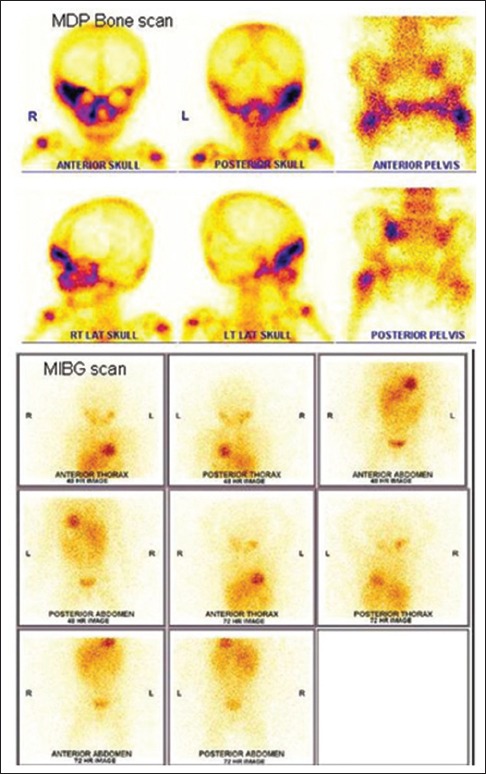
| Fig. 4 99mTechnetium methylene diphosphonate bone and 131I MIBG scan in a 16-month-old child with Stage IV neuroblastoma. Bone scan reveals multiple sites of bone metastases (right supra orbital and zygomatic bones, right maxilla, left sacro iliac joint, and left femoral trochanter) while metaiodobenzyl guanidine scan is negative
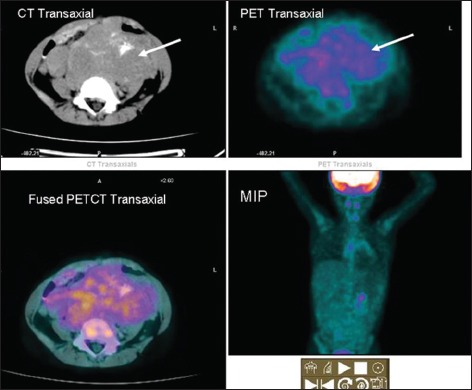
| Fig. 5 Heterogenous mildly increased fluorodeoxyglucose noted in a large primary neuroblastoma with specks of intratumoral calcification

| Fig. 6 FDG PET/CT images in a 15-year-old girl suspected to have recurrent glioma. Fused FDG PET/CT transaxial images clearly demarcate the tumor site from surrounding edema
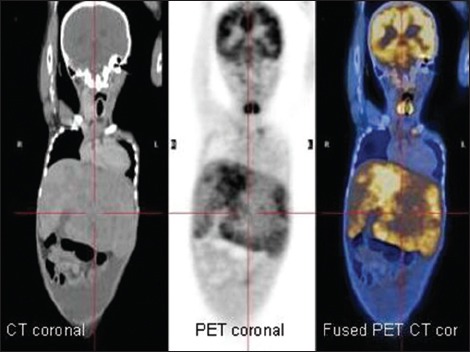
| Fig. 7 Fluorodeoxyglucose positron emission tomography/computed tomography images of a 5-year-old child with histologically proven hepatoblastoma showing variable fluorodeoxyglucose uptake (coronal sections)