Molecular MRD Assessment in Acute Myeloid Leukemias
CC BY 4.0 · Indian J Med Paediatr Oncol 2023; 44(06): 566-577
DOI: DOI: 10.1055/s-0043-1762921
Abstract
Detection of measurable residual disease (MRD) is of significant value in the management of acute myeloid leukemia (AML) patients. Along with multicolor flowcytometry (MFC), molecular techniques form an integral tool in AML MRD detection. Multiple studies have reiterated the role of molecular MRD evaluation in AML at defined timepoints during the course of therapy, helping in risk stratification, prediction of relapse, and as guide for pre-emptive therapy. The latest World Health Organization (WHO) classification (WHO-HEME5) has refined the classification of AML bringing forth newer entities defined by molecular abnormalities, especially fusions. AML is a clonally heterogeneous disease characterized by a spectrum of multiple molecular abnormalities including gene mutations and fusions. Accordingly, the molecular methods employed are also diverse and need robust technical standardization in clinical laboratories. Real-time quantitative polymerase chain reaction (PCR), digital PCR, and next-generation sequencing (NGS) are the major molecular platforms for AML MRD. The European LeukemiaNet (ELN) MRD Working Party consensus document recently updated in 2021 for the first time has reflected on the technical recommendations for NGS MRD in AML and stressed the value of an integrated approach. It is, therefore, desirable for physicians, scientists, and pathologists alike to thoroughly understand these molecular methods for appropriate utilization and interpretation. In this article, we discuss the various facets of molecular methods for MRD detection in AML including technical requirements, advantages, drawbacks, and applications.
Publication History
Article published online:
17 April 2023
© 2023. The Author(s). This is an open access article published by Thieme under the terms of the Creative Commons Attribution License, permitting unrestricted use, distribution, and reproduction so long as the original work is properly cited. (https://creativecommons.org/licenses/by/4.0/)
Thieme Medical and Scientific Publishers Pvt. Ltd.
A-12, 2nd Floor, Sector 2, Noida-201301 UP, India
Abstract
Detection of measurable residual disease (MRD) is of significant value in the management of acute myeloid leukemia (AML) patients. Along with multicolor flowcytometry (MFC), molecular techniques form an integral tool in AML MRD detection. Multiple studies have reiterated the role of molecular MRD evaluation in AML at defined timepoints during the course of therapy, helping in risk stratification, prediction of relapse, and as guide for pre-emptive therapy. The latest World Health Organization (WHO) classification (WHO-HEME5) has refined the classification of AML bringing forth newer entities defined by molecular abnormalities, especially fusions. AML is a clonally heterogeneous disease characterized by a spectrum of multiple molecular abnormalities including gene mutations and fusions. Accordingly, the molecular methods employed are also diverse and need robust technical standardization in clinical laboratories. Real-time quantitative polymerase chain reaction (PCR), digital PCR, and next-generation sequencing (NGS) are the major molecular platforms for AML MRD. The European LeukemiaNet (ELN) MRD Working Party consensus document recently updated in 2021 for the first time has reflected on the technical recommendations for NGS MRD in AML and stressed the value of an integrated approach. It is, therefore, desirable for physicians, scientists, and pathologists alike to thoroughly understand these molecular methods for appropriate utilization and interpretation. In this article, we discuss the various facets of molecular methods for MRD detection in AML including technical requirements, advantages, drawbacks, and applications.
Introduction
Acute myeloid leukemia (AML) constitutes approximately 1%-of all cancer cases as per the Surveillance, Epidemiology, and End Results Program (SEER) Program in the United States and considered a disease with dismal prognosis. It is one of the most common leukemias in adults and not a very uncommon leukemia in children. Over the last two decades, our understanding of disease biology and pathogenic mechanisms has improved and has resulted in newer targeted therapies in AML. Detection of the residual disease burden beyond conventional morphological techniques known as measurable residual disease (MRD) is a standard of care in leukemia patients and plays a crucial role in disease prognostication, predicting and monitoring of response. MRD positivity during complete morphological remission is known to be associated with increased relapse rates and poor survival outcomes in AML.[1] [2] In addition, MRD status is also explored for treatment decisions.
The European LeukemiaNet (ELN) MRD Working Party recommendations are widely followed for AML MRD, which were last updated in 2021.[3] The two major modalities for MRD detection in AML are multiparametric flow cytometry (MFC) and molecular MRD testing. Each of these two techniques has its merits and demerits. MFC is widely available and can be applied in almost all cases of AML. Assessment of CD34 +/CD38-leukemic stem cell burden in combination with aberrant markers by MFC has also been used for MRD detection. However, the detection of true minimal residual disease against a background of regenerative hematopoiesis and chemotherapy effects (such as immunophenotypic shifts), along with the technical and analytical expertise required, together pose practical challenge for AML MRD detection by MFC. This is further complicated by the fact that unlike in precursor B lineage acute lymphoblastic leukemia, the frequencies of leukemia-associated immunophenotypes in AML do not always permit a clear detection of the leukemic clone.
AML is a heterogeneous disease marked by the presence of numerous clones and subclones of leukemic cells harboring multiple molecular changes that undergo fluctuations during the disease course and therapy. Tracking of the molecular aberrations detected at diagnostic timepoint forms the basis of molecular MRD testing. The list of newer AML entities defined by molecular methods continues to expand, as evident in the latest World Health Organization (WHO) classification of hematolymphoid neoplasms (WHO-HEME5).[4] The implementation of molecular techniques for MRD monitoring is therefore need of the hour for hematopathology laboratories. We review the molecular methods for AML MRD considering the recent ELN AML MRD recommendations[3] and also discuss their clinical utility in this paper.
Overview of the Molecular Aberrations and Methods of Detection
Molecular studies performed at diagnostic timepoint help in the identification of molecular aberrations in most AML patients, each of which can be potentially tracked for MRD using molecular techniques. The molecular aberrations broadly include
a) Chimeric gene fusions as a result of chromosomal translocations, for example, RUNX1::RUNX1T1, CBFB::MYH11, PML::RARA
b) Insertions and deletions (Indels), for example, nucleophosmin 1 (NPM1), FLT3-internal tandem duplication (ITD)
c) Single nucleotide variants (SNVs), for example, TP53 R273H, KRAS G12D
d) Increased gene expression levels, for example, WT1, ERG, BAALC, PRAME, and MN1.[5] [6] [7] [8] [9] [10] [11]
Given the genomic heterogeneity of AML, a variety of molecular techniques are employed for monitoring MRD. These range from real-time quantitative polymerase chain reaction (RT-qPCR) and digital (dPCR) to next-generation sequencing (NGS)-based approaches. The latter include conventional “bulk” NGS (for single genes as well as multigene panels) and error corrected NGS. Other methods that have been reported in literature but not widely used for the purpose of MRD detection include chimerism studies post-transplant[12] and immunoglobulin heavy chain and T cell receptor gene rearrangements.[13] Exploratory methods for AML MRD include sequencing of cell free DNA, single cell genomics, and metabolomic profiling.[14] [15] [Fig. 1] provides an overview of the common molecular aberrations and the molecular methods used to detect AML MRD.
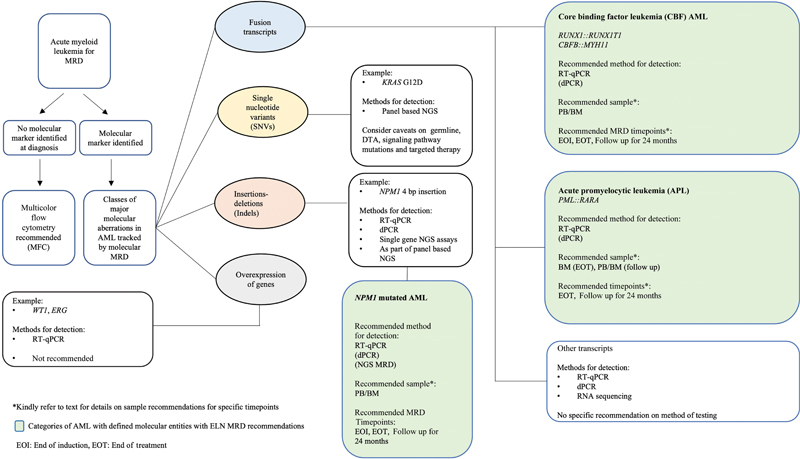
| Fig 1 : Overview of molecular aberrations in acute myeloid leukemia (AML) tracked by molecular methods and their major methods of detection along with recommended methods. BM, bone marrow; dPCR, digital PCR; MRD, measurable residual disease; NGS, next-generation sequencing; PB, peripheral blood; PC, post-consolidation; RT-qPCR, real-time quantitative polymerase chain reaction.
Needless to say, technical standardization and stringent validation in the laboratory are utmost challenging and irreplaceably required for implementation and reporting in a clinical setting.
Technical Considerations: Preanalytical
Sample Considerations
The recommended sample for molecular MRD in AML as per ELN 2021 recommendations is either bone marrow (BM) or peripheral blood (PB). The volume of draw for BM aspirate should be up to 5 mL, while a volume of more than or equal to 10 mL can be required when using PB.[3] In addition, the white cell count and the assay to be performed also need to be considered while determining the required volume of sample drawn. First pull of marrow aspirate should be preferred to avoid hemodilution.
Although ELN recommends both ethylenediaminetetraacetic acid and heparin as the anticoagulants for sample collection, heparin is better avoided given its inhibitory effect on PCR reactions. Cell isolation techniques to reduce hemodilution by granulocytes can be used (e.g., Ficoll separation), provided that the method followed in laboratory is consistent, as leukemic cell percentage can be altered based on it.
Peripheral Blood versus Bone Marrow
The use of BM yields up to one log higher sensitivity compared to PB.[16] Multiple studies have also shown concordance between the two sample types. Recently, Skou et al reviewed literature concerning the utility of PB versus BM for molecular AML MRD and suggested that PB may be sufficient for predicting impending AML relapses.[17] The ELN 2021 MRD document, however, currently recommends the use of both samples (PB and BM) at diagnostic timepoint (as comparators) if using log reduction for MRD calculation. PB sample is preferred at post-induction (PI) timepoint in NPM1 mutated and core binding factor (CBF) AMLs, while BM sample is recommended for post-consolidation (PC) timepoint. Subsequent follow-up can either be done on PB or BM.[3]
Other Considerations
Once the sample is received by the laboratory and sample requirements are met, it is essential to confirm timepoint of testing, the diagnostic molecular results, and the current disease status on morphology. These factors can influence the choice of assay to be performed and the interpretation.
Technical Considerations: Analytical
Single Biomarker Methods
RT-qPCR and digital PCR are the two major techniques recommended for molecular MRD assessment for the detection of CBF-AML, acute promyelocytic leukemia (APL), and NPM1 mut AML.[3] For patients with no molecular abnormality identified, the recommended method remains MFC.[3]
RT-qPCR: General Considerations
RT-qPCR has been the most widely adopted method for molecular MRD assessment and involves quantitative detection using fluorescent probes designed specifically to target the regions of interest. The molecular abnormalities detected by RT-qPCR broadly include (a) fusion transcripts, (b) single gene variants and indels, and (c) genes with altered expression levels in AML.
RNA is usually the starting material for detecting fusions and differential expression of genes, while both RNA and DNA have been employed for the detection of gene mutations using RT-qPCR, for example, NPM1, FLT3-ITD, IDH1, IDH2, and DNMT3A.[18] [19] [20] [21] [22] [23] [24]
The technical requirements established by Europe Against Cancer (EAC) in 2003 remain the resort for the implementation of molecular MRD in AML by RT-qPCR.[3] [25] [26] Along with the region of interest, a house keeping gene (e.g., ABL1) is also amplified (minimum 10,000 copies) and detected using specifically designed primers and probes. The obtained copy number of target gene/ fusion transcript is then normalized against that of a housekeeping gene or the wild-type counterpart of the respective mutated gene (e.g., NPM1).
A positive RT-qPCR result is defined as amplification with cycle threshold (Ct) values below 40 in at least two of the three (triplicates) results of the sample tested, when the value of cycling threshold is 0.1.[3] [25] [26] Assay sensitivity needs to be determined especially while reporting a negative result. The sensitivity achieved by RT-qPCR ranges between 10−4 and 10−5. [Table 1] highlights the major studies where RT-qPCR has been used for the detection of molecular MRD in AML in major molecularly defined entities.
Marker |
Study |
Interpretation of results |
Sensitivity |
Highlights |
---|---|---|---|---|
Core binding factor leukemia |
Viehmann S 2003[27] |
10 copies reliably detected |
10−5 |
- 92 follow-up samples from 15 pediatric AML cases studied - 2–4 log decrease at start of consolidation in most patients - Serial monitoring technique suggested for prediction of relapse |
Perea G 2006[28] |
Ct <40> Ct <39> |
10−4 to 10−5 |
- Higher CIR for cases with >10 copies at end of treatment |
|
Corbacioglu A 2010[29] |
– |
10−3 to 10−4 |
- PC MRD in CBFB::MYH11 AML identifies patients with high relapse risk - Showed that PB can be used for monitoring in patients with negative MRD after consolidation and early follow-up |
|
Yin JA et al 2012[30] |
As per EAC criteria |
10−5 |
- PI BM MRD >3 log, BM copies >500 and PB >1000 informative for RUNX1::RUNX1T1 - PC BM MRD ≥4 log reduction associated with lower CIR, and presence of >500 RUNX1::RUNX1T1 copies predicted relapse - Presence of >10 copies of CBFB::MYH11 in PB associated with higher CIR |
|
Zhu HH 2013[31] |
As per QinYZLiJLZhuHH et al |
Not mentioned |
- Positive MRD after 2nd consolidation discriminated patients with high relapse risk |
|
Jourdan E 2013[32] |
As per EAC criteria |
Not mentioned |
- PC MRD (after 2nd consolidation) could best discriminate patients with high relapse risk |
|
Wang Y 2014[33] |
As per EAC criteria |
Not mentioned |
- Post-HSCT monitoring of RUNX1::RUNX1T1 at 1, 2, 3 months |
|
Willekens et al 2016[34] |
0.001-%- |
10−5 |
- 94 paired PB and BM samples prospectively - Two years follow-up - Serial PB MRD of RUNX1::RUNX1T1 monitoring predicted hematological relapse |
|
Acute promyelocytic leukemia |
Grimwade D 2009[35] |
As per EAC criteria |
10−4 |
- 406 patients monitored prospectively - Sequential MRD post-consolidation predicted relapse and helps guide pre-emptive therapy |
NPM1 |
Gorello P 2006[36] |
– |
10−4 to 10−5 |
- NPM1 types A and B mutations studied - NPM1 shown as stable MRD marker |
Chou WC 2007[21] |
– |
10−5 |
- DNA based study - Ten years follow-up of 194 samples from 38 AML patients - Patients with at least 2 log reduction PC showed better OS and PFS |
|
Papadaki 2009[37] |
– |
10−5 |
- RNA based - 51 patients with Type A mutation studied - Both PB and BM studied - 9.5%-relapsed cases showed loss of NPM1 mutation |
|
Schnittger S 2009[38] |
– |
10−5 |
- Paired diagnostic/relapse samples of 84 patients studied - 17 types of NPM1 mutations studied - A threshold of 0.1%-found relevant for EFS at four timepoints - No impact on relapse risk or OS |
|
Krönke J 2011[39] |
– |
10−5 to 10−6 |
- RNA-based, six types of NPM1 detected - 245 young adult patients - Cutoff of 200 copies/104 ABL copies predicted relapse |
|
Shayegi 2013[40] |
– |
10−5 |
- NPM1 types A, B, and D included - LNA clamping strategy used - MRD detectable above 1%-at CR1 significantly correlated with worse DFS and OS |
|
Ivey A 2016[19] |
As per EAC criteria |
10−4 |
- 2,569 samples from 346 patients. - MRD positivity associated with inferior 3-year survival and higher relapse rates |
Study |
Method |
Interpretation of results |
Highlights |
---|---|---|---|
Klco et al 2015[55] |
- Targeted amplicon-based sequencing - Ion Torrent platform |
- VAF of 2.5%-and 1%-studied as cut offs |
- Day 30 remission FFPE BM biopsy samples of 25 patients. - Increased relapse risk and reduced OS in MRD positive cases |
Getta et al 2017[56] |
- Amplicon capture-based NGS - 28 gene panel |
- VAF <5> |
- Pre-HSCT MRD studied in 47 patients - Presence of MRD shown to be associated with post-transplant relapse and survival - Compared with MFC MRD |
Jongen-Lavrencic 2018[57] |
- Targeted NGS - 54 genes |
- Maximum sensitivity of 0.02%- achieved |
- 482 patients - PI MRD positivity associated with increased rates of relapse, RFS and OS - DTA mutations excluded - Compared with MFC MRD |
Kim et al 2018[58] |
- Targeted NGS - 84 gene panel - Mean on target coverage 1725.6x |
- Minimum VAF of 0.02%-called |
- 104 patients - Post-HSCT day 21 VAF of ≥ 0.2%-observed to be associated with increased relapse and worse 3 years OS |
Morita et al 2018[59] |
- Haloplex HS molecular barcode sequencing - 32 genes |
- Mutation clearance defined as per VAF at CR (2.5%, 1%, undetectable) |
- 131 patients - Cases with VAF less than 1%-and undetectable mutations at PI had better EFS, OS, and lower CIR at median follow-up of 35.2 months - CHIP mutations found noninformative |
Thol et al 2018[16] |
- Targeted UMI based error corrected sequencing - 24 genes |
- Sensitivity of <0> |
- 96 cases in CR pre-transplant - MRD positivity shown to be associated with higher CIR and lower PFS at median follow-up of 6.2 years - Comparable results in PB and BM |
Press et al 2019[60] |
- Ampliseq panel of 42 gene - PGM sequencer platform - Mean coverage 1900x |
- Lower limit of detection (LLOD) of 0.24%- |
- 42 cases pre-HSCT (≤27 days) - MRD positivity shown to independently predict relapse and associated with significantly shortened PFS |
Hourigan et al 2019[61] |
- Ultra-deep, error-corrected sequencing - 13 gene panel |
- 0.001% VAF |
- 190 cases - RIC conditioning associated with increased relapse rates decreased OS and compared to MAC in pre-transplant NGS MRD positive cases |
Balagopal et al 2019[62] |
- Hybrid capture based error corrected targeted NGS incorporating UMIs - 22 genes targeted |
- VAF of 0.1%-for SNVs and 0.001%-for indels |
- Retrospective analysis of 30 relapsed patients post-HSCT using pre recurrence samples - NGS MRD effective for surveillance post-HSCT to identify relapse - NGS more useful than STR PCR and MFC - Suggest that CHIP mutations can be used post-transplant |
Onecha et al 2019[63] |
- 32 genes - ≥ 1000000 read depth - Ion Torrent platform |
- 10 − 4 (SNVs) 10 − 5 (indels) |
- Samples from 63 patients studied at PI and PC timepoints - CHIP mutations excluded - Sensitivity compared to dPCR |
Patkar et al 2021[64] |
- 34 gene panel - smMIPS based - FLT3-ITD studied using gene specific NGS assays - Median coverage 14,728x |
- 0.05%-for SNVs and 0.03%-for FLT3-ITD 0.002%- |
- 201 patients - Presence of positive NGS-MRD predictive of inferior OS and RFS for PI and PC timepoints - Median follow-up of 42.3 months - Compared with MFC MRD |

| Figure 2:llustration of error correction in next-generation sequencing (A) and overview of error correction techniques applied for measurable residual disease detection in NGS (B).[65] [66] [67]PCR, polymerase chain reaction; smMIPS, single molecular inversion probe system.
Library Preparation
Library preparation involves sample processing steps to make the sample ready for sequencing, and broadly includes fragmentation, end repair, A-tailing, and adaptor ligation. Modifications in library preparation are required for MRD detection in order to achieve higher sensitivity and correct variant calls. Incorporation of unique molecular identifiers (UMI) is the most widely used technique that can be performed using commercial or laboratory developed methods ([Fig. 2B]). Broadly, the methods for UMI incorporation are either single molecular inversion probe system (smMIPS) based or PCR based. Further, the UMIs can be incorporated on single strand or both strands (duplex), with duplex method providing higher error correction.[65] [66] We have demonstrated the utility of error-corrected NGS using in house laboratory developed 34- gene panel using smMIPS and single gene assays for NPM1 and FLT3-ITD.[64]
LOD for NGS Assays
Results of NGS are expressed in terms of VAF, defined by proportion of reads containing the mutant allele out of the total reads obtained for that particular locus. The LOD for conventional NGS methods applied at diagnostic timepoints ranges from 2 to 5%,- which lowers down to the desirable 0.1 to 0.5%-when error correction is applied.[66]
Sequencing Depth
Achievement of high sensitivity in MRD requires a higher sequencing depth (>20,000x) while using non-error corrected sequencing.[3] [66] A read depth high enough to discriminate true call from background noise should be targeted.[3] Deeper approach is usually possible using a limited set of genes and tracking mutations detected earlier in the patient. Use of smaller panels with deeper sequencing leads to compromise on “breadth” leading to chance of missing mutations: (a) present at diagnostic timepoint but not targeted in the MRD panel and (b) newer mutations that could potentially lead to relapse.
Markers for Panel-Based NGS-MRD
Potentially every gene mutation detected at diagnostic timepoint can be targeted for MRD detection by targeted NGS. A panel of 23 commonly mutated genes in AML has been suggested by ELN.[3] Four important considerations while choosing markers for analysis of NGS MRD are:
(i) DTA mutations (DNMT3A, TET2, ASXL1) associated with clonal hematopoiesis of indeterminate potential (CHIP): The mutations associated with CHIP should not be considered for MRD analysis as they are known to persist post-remission and also may not be part of leukemic clone.[57] [64] [68] If DTA mutations are the only detected mutations at the diagnostic time point, MFC based MRD or PCR-based MRD should be applied.[3]
(ii) Germline mutations: Mutations in genes known to be associated with germline predisposition to myeloid malignancies example, CEBPA, DDX41, are detected at near heterozygous VAF (∼50%-) at baseline and persist at further timepoints. These variants are noninformative and should not be used as MRD markers.[3] A potential exception to this situation could be post allogenic hematopoietic stem cell transplant (allo-HCT)[65]
(iii) Signaling pathway genes: Mutations in the signaling pathway, for example, FLT3-ITD, FLT3-TKD, KIT, KRAS, NRAS, are likely secondary events in leukemogenesis and are mostly subclonal. When detected at MRD timepoint, they represent true MRD positivity; however, their absence in isolation should not be taken as evidence of MRD negativity.[3]
(iv) Targeted therapy: When performing MRD in a patient who has received targeted therapy, for example FLT3 inhibitors or IDH1/2 inhibitors, a marker apart from that targeted should also be included in the MRD analysis.[3]
Single Gene NGS: NPM1 and FLT3-ITD
The limitations of RT-qPCR (as discussed in previous sections) are addressed to a large extent with the use of NGS for NPM1 MRD detection as patient-specific primers are not required.[41] [42] [69] The FLT3-ITD mutations in AML involve insertions in the exon 14 (NM_004119) of variable length affecting the juxta membrane domain of the FLT3 gene. With the widespread use of targeted therapy in the treatment of FLT3-ITD AML, monitoring forms an essential part of patient management. Sensitive PCR-based assays would require patient specific FLT3-ITD primers limiting its practical utility. Additionally, the variable length of ITDs also limits the use of conventional NGS algorithms.[70] Specific NGS assays for monitoring of FLT3-ITD targeting the exon 14 have been designed and implemented.[64] [70] [71] [72] As emphasized in a previous section, ELN recommends integration of an additional marker in MRD analysis while interpretating a negative FLT3-ITD MRD, owing to the unstable and possible subclonal nature of the FLT3-ITD mutations.[73] [74]
Defining MRD Positivity by NGS
Presence of a mutation at VAF more than or equal to 0.1%-provisionally defines NGS-MRD positivity. As a VAF lower than 0.1%-might also be possibly associated with adverse outcome, it is recommended to be reported as MRD-LL (low level).[3]
Informatics Considerations
Bioinformatics involves computational methods to process sequencing raw data and derive results for analysis. Calculation of error rate, reducing false positive calls, and calculation of sensitivity are crucial elements in bioinformatics in context of AML MRD. Derivation of background error rate involves calculation of the largest VAF of nucleotides that flank the target (excluding primer sequence). Sensitivity for a sample is calculated by dividing the mean background error by number of read families (or reads) expressed as percentage.[3]
There are currently no uniform recommendations on the use of specific bioinformatics pipeline, and this area still requires harmonized efforts for standardization. It is important to note that tools for detection of indels are required to be different from the regular alignment tools for SNVs.
ELN recommends that for a sample to be evaluable when error-corrected sequencing approach is used, at least 10,000 read families and more than 10 mutant reads should be present. At least three reads should be present in each read family. For a sample to be evaluable for non-error-corrected sequencing approaches, the total reads and mutant reads recommended are more than or equal to 60,000 and more than 60, respectively. When background error correction is applied, MRD positivity is defined by a VAF greater than the sum of mean background error and 3x standard deviation of the background error. There can be other methods to define MRD positivity.[3]
Targeted RNA Sequencing
As previously discussed, RT-qPCR forms the gold standard for molecular MRD detection of common fusion transcripts, while the follow-up of uncommon fusions relies on relative quantification. Targeted RNA sequencing using UMI correction forms a promising alternative for molecular MRD of fusion transcripts possible in a single assay for multiple fusion transcripts.[75] Dillon et al demonstrated a sensitivity of 1 in 100,000 for detection of molecular MRD in AML using UMI-based multiplexed RNA sequencing assay.[76] The utility of RNA sequencing has also been explored by Kim et al in cases of CBF-AML, where they demonstrated that the reduction in disease burden was comparable between RNA sequencing and RT-qPCR.[77] However, unlike DNA-based NGS MRD, the literature on RNA sequencing based MRD for follow-up of fusion transcripts is still sparse and requires further exploration.
Limitations of NGS
While high sensitivity, throughput, and wider applicability give NGS an edge over other techniques, lack of standardization across laboratories and establishment of clinically relevant cutoffs are some issues which need addressal. Other factors influencing interpretation of NGS MRD include possibility of finding certain gene mutations in healthy individuals, and the fact that some genetic abnormalities can persist at low levels while patient is clinically stable can pose interpretation problems while reporting.[45] [78] Consequently, NGS as a standalone technique is not currently recommended by ELN.[3]
Clinical Practice Considerations
Definitions of Response
The recommended definitions of response and relapse based on MRD are summarized in [Table 3].[3]
Response category |
Response criteria |
---|---|
CR with negative MRD (CR MRD-) |
- Complete morphologic remission (CR) and - MRD negativity by all MRD technologies used a. BM MFC-MRD negative (if MFC-MRD was used). b. MRD negative by RT-qPCR in BM (or in PB after cycle 2 for NPM1 and CBF-MRD) (if qPCR-MRD used). c. MRD negative by NGS in BM (if NGS-MRD used). |
CR with positive MRD (CR MRD + ) |
1. Morphological CR and 2. MRD positivity in PB and/or BM either by MFC or NGS-MRD or qPCR-MRD positive. |
CR with molecular MRD detection at low level (CR-MRD-LL) |
- Morphologic CR and - Presence of PB and/or BM MRD detectable by molecular methods at low level (qPCR for NPM1 <2> |
MRD relapse |
1. Conversion of a negative MRD result to positive by any MRD method or 2. increase in MRD copy numbers ≥1 log10 between any 2 positive samples in the cases with CR-MRD-LL monitored by qPCR. 3. Above results should be repeated on a second sample and confirmed, BM preferably. |
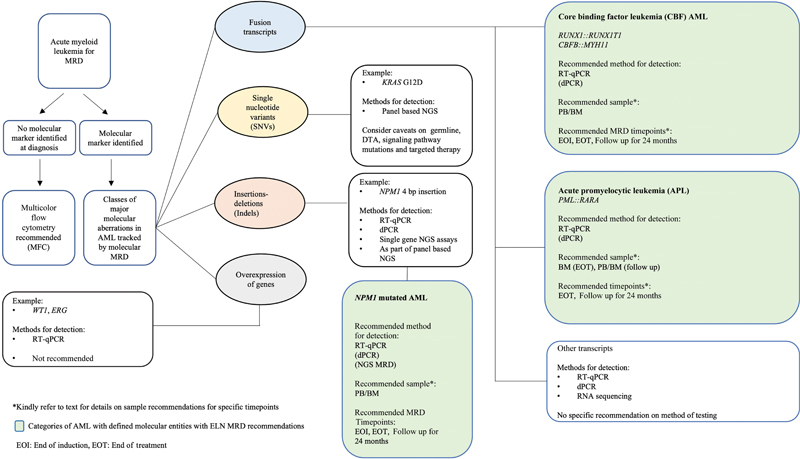
| Fig 1 : Overview of molecular aberrations in acute myeloid leukemia (AML) tracked by molecular methods and their major methods of detection along with recommended methods. BM, bone marrow; dPCR, digital PCR; MRD, measurable residual disease; NGS, next-generation sequencing; PB, peripheral blood; PC, post-consolidation; RT-qPCR, real-time quantitative polymerase chain reaction.

| Figure 2:llustration of error correction in next-generation sequencing (A) and overview of error correction techniques applied for measurable residual disease detection in NGS (B).[65] [66] [67]PCR, polymerase chain reaction; smMIPS, single molecular inversion probe system.
References
- 1 Kantarjian H, Kadia T, DiNardo C. et al. Acute myeloid leukemia: current progress and future directions. Blood Cancer J 2021; 11 (02) 41
- 2 Patkar N, Kakirde C, Bhanshe P. et al. Utility of immunophenotypic measurable residual disease in adult acute myeloid leukemiareal-world context. Front Oncol 2019; 9: 450
- 3 Heuser M, Freeman SD, Ossenkoppele GJ. et al. 2021 Update on MRD in acute myeloid leukemia: a consensus document from the European LeukemiaNet MRD Working Party. Blood 2021; 138 (26) 2753-2767
- 4 Khoury JD, Solary E, Abla O. et al. The 5th edition of the World Health Organization Classification of Haematolymphoid Tumours: Myeloid and Histiocytic/Dendritic Neoplasms. Leukemia 2022; 36 (07) 1703-1719
- 5 Rautenberg C, Lauseker M, Kaivers J. et al. Prognostic impact of pretransplant measurable residual disease assessed by peripheral blood WT1-mRNA expression in patients with AML and MDS. Eur J Haematol 2021; 107 (02) 283-292
- 6 Weisser M, Kern W, Rauhut S. et al. Prognostic impact of RT-PCR-based quantification of WT1 gene expression during MRD monitoring of acute myeloid leukemia. Leukemia 2005; 19 (08) 1416-1423
- 7 Najima Y, Ohashi K, Kawamura M. et al. Molecular monitoring of BAALC expression in patients with CD34-positive acute leukemia. Int J Hematol 2010; 91 (04) 636-645
- 8 Weber S, Alpermann T, Dicker F. et al. BAALC expression: a suitable marker for prognostic risk stratification and detection of residual disease in cytogenetically normal acute myeloid leukemia. Blood Cancer J 2014; 4 (01) e173
- 9 Lazzarotto D, Candoni A. The role of Wilms' Tumor Gene (WT1) expression as a marker of minimal residual disease in acute myeloid leukemia. J Clin Med 2022; 11 (12) 3306
- 10 Qin Y, Zhu H, Jiang B. et al. Expression patterns of WT1 and PRAME in acute myeloid leukemia patients and their usefulness for monitoring minimal residual disease. Leuk Res 2009; 33 (03) 384-390
- 11 Jentzsch M, Bill M, Grimm J. et al. Prognostic impact of blood MN1 copy numbers before allogeneic stem cell transplantation in patients with acute myeloid leukemia. HemaSphere 2019; 3 (01) e167
- 12 Tsirigotis P, Byrne M, Schmid C. et al. Relapse of AML after hematopoietic stem cell transplantation: methods of monitoring and preventive strategies. A review from the ALWP of the EBMT. Bone Marrow Transplant 2016; 51 (11) 1431-1438
- 13 Zhong L, Chen J, Huang X, Li Y, Jiang T. Monitoring immunoglobulin heavy chain and T-cell receptor gene rearrangement in cfDNA as minimal residual disease detection for patients with acute myeloid leukemia. Oncol Lett 2018; 16 (02) 2279-2288
- 14 Panuzzo C, Jovanovski A, Ali MS, Cilloni D, Pergolizzi B. Revealing the mysteries of acute myeloid leukemia: from quantitative PCR through next-generation sequencing and systemic metabolomic profiling. J Clin Med 2022; 11 (03) 483
- 15 Ediriwickrema A, Aleshin A, Reiter JG. et al. Single-cell mutational profiling enhances the clinical evaluation of AML MRD. [published correction appears in Blood Adv. 2020 Apr 14;4(7):1218] Blood Adv 2020; 4 (05) 943-952
- 16 Thol F, Gabdoulline R, Liebich A. et al. Measurable residual disease monitoring by NGS before allogeneic hematopoietic cell transplantation in AML. Blood 2018; 132 (16) 1703-1713
- 17 Skou AS, Juul-Dam KL, Ommen HB, Hasle H. Peripheral blood molecular measurable residual disease is sufficient to identify patients with acute myeloid leukaemia with imminent clinical relapse. Br J Haematol 2021; 195 (03) 310-327
- 18 Dvorakova D, Racil Z, Jeziskova I. et al. Monitoring of minimal residual disease in acute myeloid leukemia with frequent and rare patient-specific NPM1 mutations. Am J Hematol 2010; 85 (12) 926-929
- 19 Ivey A, Hills RK, Simpson MA. et al; UK National Cancer Research Institute AML Working Group. Assessment of minimal residual disease in standard-risk AML. N Engl J Med 2016; 374 (05) 422-433
- 20 Balsat M, Renneville A, Thomas X. et al. Postinduction minimal residual disease predicts outcome and benefit from allogeneic stem cell transplantation in acute myeloid leukemia with NPM1 mutation: a study by the Acute Leukemia French Association Group. J Clin Oncol 2017; 35 (02) 185-193
- 21 Chou WC, Tang JL, Wu SJ. et al. Clinical implications of minimal residual disease monitoring by quantitative polymerase chain reaction in acute myeloid leukemia patients bearing nucleophosmin (NPM1) mutations. Leukemia 2007; 21 (05) 998-1004
- 22 Abdelhamid E, Besbes S, Renneville A. et al. Minimal residual disease assessment of IDH1/2 mutations in acute myeloid leukemia by LNA-RQ-PCR. Tunis Med 2016; 94 (03) 190-197
- 23 Petrova L, Vrbacky F, Lanska M, Zavrelova A, Zak P, Hrochova K. IDH1 and IDH2 mutations in patients with acute myeloid leukemia: Suitable targets for minimal residual disease monitoring?. [published correction appears in Clin Biochem. 2019 Jan;63:161] Clin Biochem 2018; 61: 34-39
- 24 Gaidzik VI, Weber D, Paschka P. et al; German-Austrian Acute Myeloid Leukemia Study Group (AMLSG). DNMT3A mutant transcript levels persist in remission and do not predict outcome in patients with acute myeloid leukemia. Leukemia 2018; 32 (01) 30-37
- 25 Beillard E, Pallisgaard N, van der Velden VH. et al. Evaluation of candidate control genes for diagnosis and residual disease detection in leukemic patients using ‘real-time’ quantitative reverse-transcriptase polymerase chain reaction (RQ-PCR) - a Europe against cancer program. Leukemia 2003; 17 (12) 2474-2486
- 26 Gabert J, Beillard E, van der Velden VH. et al. Standardization and quality control studies of ‘real-time’ quantitative reverse transcriptase polymerase chain reaction of fusion gene transcripts for residual disease detection in leukemia - a Europe Against Cancer program. Leukemia 2003; 17 (12) 2318-2357
- 27 Viehmann S, Teigler-Schlegel A, Bruch J, Langebrake C, Reinhardt D, Harbott J. Monitoring of minimal residual disease (MRD) by real-time quantitative reverse transcription PCR (RQ-RT-PCR) in childhood acute myeloid leukemia with AML1/ETO rearrangement. Leukemia 2003; 17 (06) 1130-1136
- 28 Perea G, Lasa A, Aventín A. et al; Prognostic value of minimal residual disease (MRD) in acute myeloid leukemia (AML) with favorable cytogenetics [t(8;21) and inv(16)]. Leukemia 2006; 20 (01) 87-94
- 29 Corbacioglu A, Scholl C, Schlenk RF. et al. Prognostic impact of minimal residual disease in CBFB-MYH11-positive acute myeloid leukemia. J Clin Oncol 2010; 28 (23) 3724-3729
- 30 Yin JA, O'Brien MA, Hills RK, Daly SB, Wheatley K, Burnett AK. Minimal residual disease monitoring by quantitative RT-PCR in core binding factor AML allows risk stratification and predicts relapse: results of the United Kingdom MRC AML-15 trial. Blood 2012; 120 (14) 2826-2835
- 31 Zhu HH, Zhang XH, Qin YZ. et al. MRD-directed risk stratification treatment may improve outcomes of t(8;21) AML in the first complete remission: results from the AML05 multicenter trial. Blood 2013; 121 (20) 4056-4062
- 32 Jourdan E, Boissel N, Chevret S. et al; French AML Intergroup. Prospective evaluation of gene mutations and minimal residual disease in patients with core binding factor acute myeloid leukemia. Blood 2013; 121 (12) 2213-2223
- 33 Wang Y, Wu DP, Liu QF. et al. In adults with t(8;21)AML, posttransplant RUNX1/RUNX1T1-based MRD monitoring, rather than c-KIT mutations, allows further risk stratification. Blood 2014; 124 (12) 1880-1886
- 34 Willekens C, Blanchet O, Renneville A. et al; French AML Intergroup. Prospective long-term minimal residual disease monitoring using RQ-PCR in RUNX1-RUNX1T1-positive acute myeloid leukemia: results of the French CBF-2006 trial. Haematologica 2016; 101 (03) 328-335
- 35 Grimwade D, Jovanovic JV, Hills RK. et al. Prospective minimal residual disease monitoring to predict relapse of acute promyelocytic leukemia and to direct pre-emptive arsenic trioxide therapy. J Clin Oncol 2009; 27 (22) 3650-3658
- 36 Gorello P, Cazzaniga G, Alberti F. et al. Quantitative assessment of minimal residual disease in acute myeloid leukemia carrying nucleophosmin (NPM1) gene mutations. Leukemia 2006; 20 (06) 1103-1108
- 37 Papadaki C, Dufour A, Seibl M. et al. Monitoring minimal residual disease in acute myeloid leukaemia with NPM1 mutations by quantitative PCR: clonal evolution is a limiting factor. Br J Haematol 2009; 144 (04) 517-523
- 38 Schnittger S, Kern W, Tschulik C. et al. Minimal residual disease levels assessed by NPM1 mutation-specific RQ-PCR provide important prognostic information in AML. Blood 2009; 114 (11) 2220-2231
- 39 Krönke J, Schlenk RF, Jensen KO. et al. Monitoring of minimal residual disease in NPM1-mutated acute myeloid leukemia: a study from the German-Austrian acute myeloid leukemia study group. J Clin Oncol 2011; 29 (19) 2709-2716
- 40 Shayegi N, Kramer M, Bornhäuser M. et al; Study Alliance Leukemia (SAL). The level of residual disease based on mutant NPM1 is an independent prognostic factor for relapse and survival in AML. Blood 2013; 122 (01) 83-92 DOI: 10.1182/blood-2012-10-461749.
- 41 Salipante SJ, Fromm JR, Shendure J, Wood BL, Wu D. Detection of minimal residual disease in NPM1-mutated acute myeloid leukemia by next-generation sequencing. Mod Pathol 2014; 27 (11) 1438-1446
- 42 Patkar N, Kodgule R, Kakirde C. et al. Clinical impact of measurable residual disease monitoring by ultradeep next generation sequencing in NPM1 mutated acute myeloid leukemia. Oncotarget 2018; 9 (93) 36613-36624
- 43 Tsai CH, Tang JL, Tien FM. et al. Clinical implications of sequential MRD monitoring by NGS at 2 time points after chemotherapy in patients with AML. Blood Adv 2021; 5 (10) 2456-2466
- 44 Abdelhamid E, Preudhomme C, Helevaut N. et al. Minimal residual disease monitoring based on FLT3 internal tandem duplication in adult acute myeloid leukemia. Leuk Res 2012; 36 (03) 316-323
- 45 Ravandi F, Walter RB, Freeman SD. Evaluating measurable residual disease in acute myeloid leukemia. Blood Adv 2018; 2 (11) 1356-1366
- 46 Huggett JF, Foy CA, Benes V. et al. The digital MIQE guidelines: minimum information for publication of quantitative digital PCR experiments. Clin Chem 2013; 59 (06) 892-902
- 47 Huggett JF. dMIQE Group. The digital MIQE guidelines update: minimum information for publication of quantitative digital PCR experiments for 2020. [published correction appears in Clin Chem. 2020 Nov 1;66(11):1464] Clin Chem 2020; 66 (08) 1012-1029
- 48 Mencia-Trinchant N, Hu Y, Alas MA. et al. Minimal residual disease monitoring of acute myeloid leukemia by massively multiplex digital PCR in patients with NPM1 mutations. J Mol Diagn 2017; 19 (04) 537-548
- 49 Ferret Y, Boissel N, Helevaut N. et al. Clinical relevance of IDH1/2 mutant allele burden during follow-up in acute myeloid leukemia. A study by the French ALFA group. Haematologica 2018; 103 (05) 822-829
- 50 Winters A, Goosman M, Stevens BM. et al. Tracking of AM associated mutations via droplet digital PCR is predictive of outcomes post-transplant. Blood 2018; 132: 2138
- 51 Brunetti C, Anelli L, Zagaria A. et al. Droplet digital PCR is a reliable tool for monitoring minimal residual disease in acute promyelocytic leukemia. J Mol Diagn 2017; 19 (03) 437-444
- 52 Brambati C, Galbiati S, Xue E. et al. Droplet digital polymerase chain reaction for DNMT3A and IDH1/2 mutations to improve early detection of acute myeloid leukemia relapse after allogeneic hematopoietic stem cell transplantation. Haematologica 2016; 101 (04) e157-e161
- 53 Bill M, Grimm J, Jentzsch M. et al. Digital droplet PCR-based absolute quantification of pre-transplant NPM1 mutation burden predicts relapse in acute myeloid leukemia patients. Ann Hematol 2018; 97 (10) 1757-1765
- 54 Grassi S, Guerrini F, Ciabatti E. et al. Digital droplet PCR is a specific and sensitive tool for detecting IDH2 mutations in acute myeloid leukemia patients. Cancers (Basel) 2020; 12 (07) 30
- 55 Klco JM, Miller CA, Griffith M. et al. Association Between Mutation Clearance After Induction Therapy and Outcomes in Acute Myeloid Leukemia. JAMA 2015; 314 (08) 811-822
- 56 Getta BM, Devlin SM, Levine RL. et al. Multicolor Flow Cytometry and Multigene Next-Generation Sequencing Are Complementary and Highly Predictive for Relapse in Acute Myeloid Leukemia after Allogeneic Transplantation. Biol Blood Marrow Transplant 2017; 23 (07) 1064-1071
- 57 Jongen-Lavrencic M, Grob T, Hanekamp D. et al. Molecular Minimal Residual Disease in Acute Myeloid Leukemia. N Engl J Med 2018; 378 (13) 1189-1199
- 58 Kim T, Moon JH, Ahn JS. et al. Next-generation sequencing-based posttransplant monitoring of acute myeloid leukemia identifies patients at high risk of relapse. Blood 2018; 132 (15) 1604-1613
- 59 Morita K, Kantarjian HM, Wang F. et al. Clearance of Somatic Mutations at Remission and the Risk of Relapse in Acute Myeloid Leukemia. J Clin Oncol 2018; 36 (18) 1788-1797
- 60 Press RD, Eickelberg G, Froman A. et al. Next-generation sequencing-defined minimal residual disease before stem cell transplantation predicts acute myeloid leukemia relapse. Am J Hematol 2019; 94 (08) 902-912
- 61 Hourigan CS, Dillon LW, Gui G. et al. Impact of Conditioning Intensity of Allogeneic Transplantation for Acute Myeloid Leukemia With Genomic Evidence of Residual Disease. J Clin Oncol 2020; 38 (12) 1273-1283
- 62 Balagopal V, Hantel A, Kadri S. et al. Measurable residual disease monitoring for patients with acute myeloid leukemia following hematopoietic cell transplantation using error corrected hybrid capture next generation sequencing. PLoS One 2019; 14 (10) e0224097
- 63 Onecha E, Linares M, Rapado I. et al. A novel deep targeted sequencing method for minimal residual disease monitoring in acute myeloid leukemia. Haematologica 2019; 104 (02) 288-296
- 64 Patkar N, Kakirde C, Shaikh AF. et al. Clinical impact of panel-based error-corrected next generation sequencing versus flow cytometry to detect measurable residual disease (MRD) in acute myeloid leukemia (AML). Leukemia 2021; 35 (05) 1392-1404
- 65 Ghannam J, Dillon LW, Hourigan CS. Next-generation sequencing for measurable residual disease detection in acute myeloid leukaemia. Br J Haematol 2020; 188 (01) 77-85
- 66 Yoest JM, Shirai CL, Duncavage EJ. Sequencing-based measurable residual disease testing in acute myeloid leukemia. Front Cell Dev Biol 2020; 8: 249
- 67 Vonk CM, Al Hinai ASA, Hanekamp D, Valk PJM. Molecular minimal residual disease detection in acute myeloid leukemia. Cancers (Basel) 2021; 13 (21) 5431
- 68 Hasserjian RP, Steensma DP, Graubert TA, Ebert BL. Clonal hematopoiesis and measurable residual disease assessment in acute myeloid leukemia. Blood 2020; 135 (20) 1729-1738
- 69 Ritterhouse LL, Parilla M, Zhen CJ. et al. Clinical validation and implementation of a measurable residual disease assay for NPM1 in acute myeloid leukemia by error-corrected next-generation sequencing. Mol Diagn Ther 2019; 23 (06) 791-802
- 70 Levis MJ, Perl AE, Altman JK. et al. A next-generation sequencing-based assay for minimal residual disease assessment in AML patients with FLT3-ITD mutations. Blood Adv 2018; 2 (08) 825-831
- 71 Bibault JE, Figeac M, Hélevaut N. et al. Next-generation sequencing of FLT3 internal tandem duplications for minimal residual disease monitoring in acute myeloid leukemia. Oncotarget 2015; 6 (26) 22812-22821
- 72 Blätte TJ, Schmalbrock LK, Skambraks S. et al. getITD for FLT3-ITD-based MRD monitoring in AML. Leukemia 2019; 33 (10) 2535-2539
- 73 Shih LY, Huang CF, Wu JH. et al. Internal tandem duplication of FLT3 in relapsed acute myeloid leukemia: a comparative analysis of bone marrow samples from 108 adult patients at diagnosis and relapse. Blood 2002; 100 (07) 2387-2392
- 74 Garg M, Nagata Y, Kanojia D. et al. Profiling of somatic mutations in acute myeloid leukemia with FLT3-ITD at diagnosis and relapse. Blood 2015; 126 (22) 2491-2501
- 75 Patkar N, Bhanshe P, Rajpal S. et al. NARASIMHA: novel assay based on targeted RNA sequencing to identify ChiMeric gene fusions in hematological malignancies. Blood Cancer J 2020; 10 (05) 50
- 76 Dillon LW, Hayati S, Roloff GW. et al. Targeted RNA-sequencing for the quantification of measurable residual disease in acute myeloid leukemia. Haematologica 2019; 104 (02) 297-304
- 77 Kim T, Moon JH, Ahn JS. et al. RNA sequencing as an alternative tool for detecting measurable residual disease in core-binding factor acute myeloid leukemia. Sci Rep 2020; 10 (01) 20119
- 78 Zhou Y, Wood BL. Methods of detection of measurable residual disease in AML. Curr Hematol Malig Rep 2017; 12 (06) 557-567
- 79 Döhner H, Wei AH, Appelbaum FR. et al. Diagnosis and management of AML in adults: 2022 recommendations from an international expert panel on behalf of the ELN. Blood 2022; 140 (12) 1345-1377
- 80 Short NJ, Zhou S, Fu C. et al. Association of measurable residual disease with survival outcomes in patients with acute myeloid leukemia: a systematic review and meta-analysis. JAMA Oncol 2020; 6 (12) 1890-1899
- 81 Aitken MJL, Ravandi F, Patel KP, Short NJ. Prognostic and therapeutic implications of measurable residual disease in acute myeloid leukemia. J Hematol Oncol 2021; 14 (01) 137
- 82 Short NJ, Patel KP, Albitar M. et al. Targeted next-generation sequencing of circulating cell-free DNA vs bone marrow in patients with acute myeloid leukemia. Blood Adv 2020; 4 (08) 1670-1677
- 83 Nakamura S, Yokoyama K, Shimizu E. et al. Prognostic impact of circulating tumor DNA status post-allogeneic hematopoietic stem cell transplantation in AML and MDS. Blood 2019; 133 (25) 2682-2695
- 84 Rausch C, Rothenberg-Thurley M, Buerger SA. et al. Double dropoff droplet digital PCR: a novel, versatile tool for mutation screening and residual disease monitoring in acute myeloid leukemia using cellular or cell-free DNA. J Mol Diagn 2021; 23 (08) 975-985